Looking beyond traditional oncogenic pathways to break cancer resistance
Posted: 25 September 2024 | Stefanie Flückiger-Mangual (TOLREMO Therapeutics) | No comments yet
Drug resistance is the leading cause of poor clinical outcomes for cancer patients undergoing targeted therapies. While current treatments are designed to inhibit targets along established oncogenic signalling cascades, a new, exciting approach is to address non-oncogene-related resistance mechanisms that operate in parallel to the “classic” oncogene pathways. Blocking transcriptional re-programming by inhibiting the bromodomain of the clinically validated target CBP/p300, for example, could provide a novel approach to preventing resistance and boosting the efficacy of existing cancer therapies. Stefanie Flückiger-Mangual, CEO and Founder of TOLREMO discusses this further.
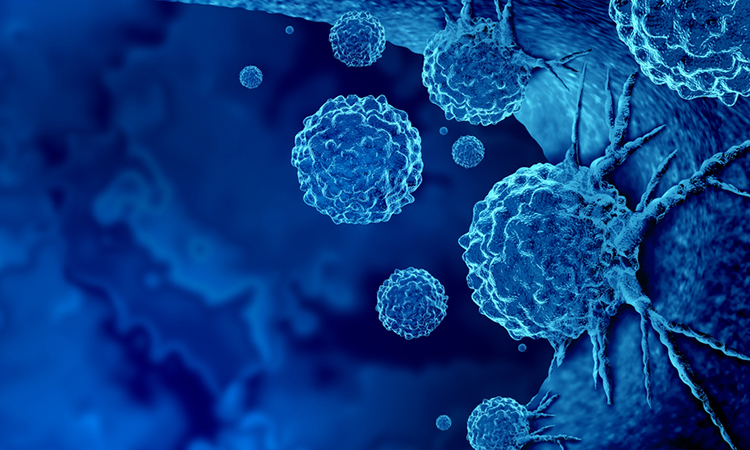
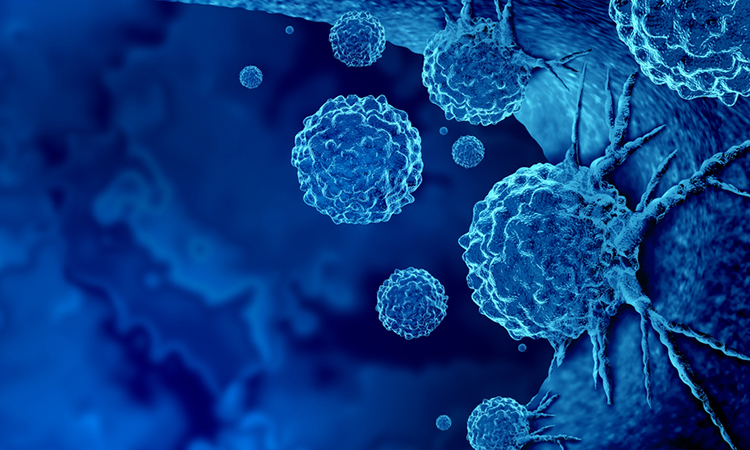
Targeted cancer therapies and treatment resistance mechanisms
Targeted cancer therapies such as epidermal growth factor receptor (EGFR), Kirsten rat sarcoma virus (KRAS), or V-Raf Murine Sarcoma Viral Oncogene Homolog B (BRAF) inhibitors have made a significant positive impact in the treatment of solid tumours, specifically for patients exhibiting oncogenic mutations. While these approaches often produce encouraging initial results, the development of drug resistance remains a major obstacle for long-term patient survival.
Most targeted cancer therapies used today operate by inhibiting targets along well-known oncogenic signalling cascades. These are activated by oncogenic driver mutations in proto-oncogenes such as EGFR, KRAS, or BRAF. The reactivation of oncogenic signalling upstream or downstream of the driving oncogene is a well-studied source of resistance to targeted cancer therapies. Classically, rational drug combinations are designed to target two nodes in the same oncogenic signalling pathway. BRAF and downstream mitogen-activated extracellular signal-regulated kinase (MEK) inhibitors are combined to treat BRAF-mutated melanoma, for example. Similarly, KRAS and upstream EGFR inhibitors work together to fight KRAS-mutated colorectal cancer.
However, growing clinical evidence points to a harsh reality: resistance to targeted therapies also occurs due to mechanisms that run in parallel to the primary oncogenic signalling cascade, which renders classical combination strategies ineffective. Non-oncogene-dependent resistance can arise because of epigenetic changes and phenotypic plasticity. These can support the survival and proliferation of malignant cells despite the presence of a targeted cancer therapy, and thereby contribute to poor and short-lived therapeutic responses.1,2,3 For example, epithelial-to-mesenchymal transition (EMT) describes the plastic adoption of an invasive cell state that is important in the physiology of normal tissues for processes such as wound healing, but is also hijacked by cancer cells for invasion and metastasis formation. Moreover, cancer cells that reactivate transcriptional stemness pathways, which are normally reserved for the developing organism, are able to tolerate therapeutic insults better than cells in a more differentiated state. Similarly, mesenchymal cell states have been described to be more resistant to cancer therapies than epithelial cell states.4 Another related and well described non-oncogene resistance mechanism is the histological transformation of EGFR-mutated non-small cell lung cancer (NSCLC) to small-cell lung cancer upon treatment with an EGFR inhibitor.5 Here, the original oncogene is still present but the transdifferentiated cancer cells are less dependent on it. Together, these examples highlight the complex interplay of resistance mechanisms that tumours utilise to survive.
Investigating non-oncogene resistance as a parallel defense mechanism used by cancer cells to evade therapies could therefore present a significant opportunity to address drug resistance more comprehensively. However, this will not be straightforward, given that non-oncogene resistance is driven by complex transcriptional networks, and the key drivers that determine how these mechanisms could be targets for drug development remain elusive.
A new role for CBP/p300 in regulating non-oncogene resistance
To identify new treatment strategies that address non-oncogene resistance, the collective industry must approach drug development from new angles. At TOLREMO, we have developed a proprietary modular phenotypic screening platform that leverages transcriptional reprogramming events associated with non-genetic resistance to deliver new chemical scaffolds and identify novel resistance regulators. Applying our technology has enabled us to develop a new small molecule, TT125-802, and to assign a new role to the epigenetic regulator CBP/p300 as a novel master regulator of non-oncogene resistance.6
CREB-binding protein (CBP) and p300, two lysine acetyltransferase paralogues, are transcriptional co-activators and well-known for their involvement in oncogenic signalling, regulation of stress responses and cell fate determination. Due to their known ability to regulate oncogenic pathways in haematologic malignancies and androgen receptor signalling, these proteins have primarily been assessed for their potential as targets in anti-cancer monotherapies for multiple myeloma, lymphomas, and prostate cancer. Our innovative screening and drug development approach has allowed us to position these clinically validated targets into a new scientific and clinical framework, unlocking new treatment possibilities for patients.
Advancing CPB/p300 bromodomain inhibitors into the clinic
Leveraging our platform of phenotypic assays, we developed the novel clinical asset TT125-802. This orally available small molecule binds to the bromodomain of CBP/p300 in a highly specific manner. It inhibits their ability to bind chromatin at acetylated histone and non-histone proteins, and therefore the activation of resistance-causing transcriptional reprogramming. TT125-802 represents a completely novel class of CBP/p300 bromodomain inhibitors with a favourable, differentiated profile.
Preclinical studies in models of EGFR-mutated NSCLC, KRAS G12C-mutated NSCLC and colorectal cancer (CRC) demonstrated that TT125-802 prevents the development of resistance to EGFR inhibitors and KRAS G12C inhibitors, respectively.7 In mouse xenograft studies, TT125-802 achieved increased response rates and prolonged duration of response when combined with standard-of-care treatment. Notably, TT125-802 stabilised disease and prevented further outgrowth of tumours resistant to standard-of-care treatment. Analysis of transcriptional changes using RNA sequencing further confirmed that TT125-802 suppresses key non-oncogene resistance mechanisms including transcriptional networks around stemness, epithelial-mesenchymal transition (EMT), and transforming growth factor beta (TGFβ) signalling.
Overall, these combined data indicate that the clinical potential for CBP/p300 inhibitors goes beyond their well-studied role in the co-activation of oncogene signalling via androgen receptor (AR) in prostate cancer and myelocytomatosis oncogene (Myc), myeloblastosis (Myb) and interferon regulatory factor 4 (IRF4) in haematological malignancies. CBP/p300 also play a crucial role in the transcriptional control of non-oncogene dependencies. TT125-802 therefore offers a new treatment strategy to address cancer resistance with high therapeutic potential both as monotherapy as well as in combination with targeted therapies.
Based on these promising preclinical proof-of-concept data, TOLREMO initiated a Phase I dose-escalation study (NCT06403436) to assess the safety, PK and pharmacodynamic effects of TT125-802 as a monotherapy in patients with solid tumours who have exhausted all other available therapies.8 Preliminary data from the trial have shown good safety and tolerability for TT125-802, with confirmed preliminary target engagement in surrogate tissues such as hair follicles and peripheral blood mononuclear cells. Several patients have experienced disease stabilisation, and one NSCLC patient showed a confirmed partial response. Our next step will be to advance TT125-802 into clinical testing in combination with targeted therapies, such as KRAS, EGFR or AR inhibitors, in specific advanced solid tumour indications, to demonstrate its benefits in rationally designed combination therapies.
The future of cancer treatments – rational combinations to prevent drug resistance
Addressing non-oncogene resistance mechanisms in combination with oncogene-targeting therapies presents a novel, differentiated strategy to tackle drug resistance in cancer. This holistic approach has the potential to preventing resistance, enhancing the efficacy and durability of current standard-of-care treatments and providing lasting benefits for patients who currently achieve only poor and short-lived responses. Identifying novel targets that enable the development of more rational combination therapies is a crucial new frontier in advancing cancer treatments.
In addition to CBP/p300, targeting components of the wingless-related integration site (Wnt) signalling pathway could offer a compelling new approach to disrupt solid tumours’ ability to develop new survival strategies. Recent studies revealed that chronic treatment with sotorasib in KRAS-mutated NSCLC activated the canonical Wnt signalling pathway.9 Silencing the Wnt pathway components, Integrin Subunit Beta 4 (ITGB4) and β-catenin, significantly improved sotorasib sensitivity in resistant cancer cells. Inhibiting KDM5A, a regulator of cell proliferation, poses another interesting approach.10 KDM5A has been shown to play a role in the survival of drug-tolerant persister cancer cells, which can act as precursors for therapeutic relapse. Inhibiting lysine demethylase 5A (KDM5A) with a small molecule significantly decreased the number of persister cells across multiple cancer cell line models treated with standard chemotherapy or targeted agents. Clinical evaluation of such strategies will reveal how mechanistically complementary drug combinations can help shape the future of cancer therapy.
References
1.Jin H, Wang L, Bernards R. Rational combinations of targeted cancer therapies: background, advances and challenges. Nature Reviews Drug Discovery [Internet]. 2022 Dec 12 [cited 2024 Sep 4];22(3):213–34. Available from: https://www.nature.com/articles/s41573-022-00615-z
2.Bell CC, Gilan O. Principles and mechanisms of non-genetic resistance in cancer. British Journal of Cancer [Internet]. 2019 Dec 13 [cited 2024 Sep 4];122(4):465–72. Available from: https://www.nature.com/articles/s41416-019-0648-6
3.Marine JC, Dawson SJ, Dawson MA. Non-genetic mechanisms of therapeutic resistance in cancer. Nature Reviews Cancer [Internet]. 2020 Dec 1 [cited 2024 Sep 4];20(12):743–56. Available from: https://www.nature.com/articles/s41568-020-00302-4
4.Adachi Y, Kimura R, Hirade K, Ebi H. Escaping KRAS: Gaining Autonomy and Resistance to KRAS Inhibition in KRAS Mutant Cancers. Cancers [Internet]. 2021 Oct 11 [cited 2024 Sep 4];13(20):5081. Available from: https://www.mdpi.com/2072-6694/13/20/5081
5.Passaro A, Jänne PA, Mok T, Peters S. Overcoming therapy resistance in EGFR-mutant lung cancer. Nature Cancer [Internet]. 2021 Apr [cited 2024 Sep 4];2(4):377–91. Available from: https://www.nature.com/articles/s43018-021-00195-8
6.Gruber D, Charles-Henry Fabritius, Bohnacker T, Schwill M, Laudato S, Herrador R, et al. Abstract 5891: An image-based phenotypic screen identified CBP/p300 as new cancer drug resistance target and enabled the development of the clinical candidate TT125-802. Cancer research [Internet]. 2024 Mar 22 [cited 2024 Jul 8];84(6_Supplement):5891–1. Available from: https://aacrjournals.org/cancerres/article/84/6_Supplement/5891/736149
7.Bohnacker T, Gruber D, Laudato S, Schwill M, Charles-Henry Fabritius, Herrador R, et al. Abstract 3907: Targeting adaptive resistance to EGFR and KRAS G12C inhibitors by TT125-802, a novel and specific CBP/p300 bromodomain inhibitor. Cancer Research [Internet]. 2023 Apr 4 [cited 2024 Sep 4];83(7_Supplement):3907–7. Available from: https://aacrjournals.org/cancerres/article/83/7_Supplement/3907/723207/Abstract-3907-Targeting-adaptive-resistance-to
8.Boni V, Garralda E, Colombo I, Krisztian Homicsko, Brana I, Gruber D, et al. A phase 1, first-in-human, open-label study evaluating the safety, tolerability, pharmacokinetics, and efficacy of TT125-802 in patients with advanced solid tumors. Journal of Clinical Oncology [Internet]. 2024 Jun 1 [cited 2024 Sep 4];42(16_suppl):TPS3171–1. Available from: https://ascopubs.org/doi/abs/10.1200/JCO.2024.42.16_suppl.TPS3171
9.Mohanty A, Nam A, Srivastava S, Jones J, Lomenick B, Singhal SS, et al. Acquired resistance to KRAS G12C small-molecule inhibitors via genetic/nongenetic mechanisms in lung cancer. Science Advances [Internet]. 2023 Oct 13 [cited 2024 Sep 4];9(41):eade3816. Available from: https://pubmed.ncbi.nlm.nih.gov/37831779/
10.Vinogradova M, Gehling VS, Gustafson A, Arora S, Tindell C, Wilson C, et al. An inhibitor of KDM5 demethylases reduces survival of drug-tolerant cancer cells. Nature Chemical Biology [Internet]. 2016 Jul 1 [cited 2024 Sep 4];12(7):531–8. Available from: https://www.nature.com/articles/nchembio.2085
About the author
Stefanie Flückiger-Mangual, Chief Executive Officer and Founder, TOLREMO
Related topics
Cancer research, Drug Development, Drug Discovery, Oncology, Targets
Related conditions
Cancer, Cancer Research, solid tumours
Related organisations
TOLREMO Therapeutics
Related people
Stefanie Flückiger-Mangual (TOLREMO Therapeutics)