The promise of induced pluripotent stem cells for liver disease, toxicology and drug discovery
Posted: 9 April 2015 | James Adjaye (Institute for Stem Cell Research and Regenerative Medicine), Nina Graffmann (Institute for Stem Cell Research and Regenerative Medicine), Peggy Matz (Institute for Stem Cell Research and Regenerative Medicine), Wasco Wruck (Institute for Stem Cell Research and Regenerative Medicine)
We discuss the steps required for differentiating human pluripotent stem cells into hepatocyte-like cells, and how long this process takes and the quality control measures needed…
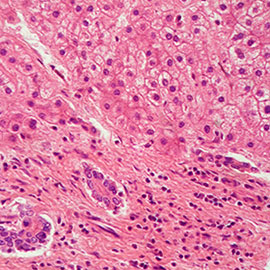
In this article, Nina Graffmann, Peggy Matz, Wasco Wruck and James Adjaye from the Institute for Stem Cell Research and Regenerative Medicine describe the three to four steps required for differentiating human pluripotent stem cells into hepatocyte-like cells, and discuss how long this process takes and the quality control measures needed. They go on to explain the drawbacks of using primary cultures of freshly-isolated human hepatocytes from donated liver biopsies, before categorising the genes that are associated with drug metabolism and moving on to discuss the use of iPSC-derived HLCs in toxicology screens to replace animal experimentation. “In general, there is an urgent requirement for in vitro models of healthy and disease-specific hepatocytes,” the authors point out.
The gold standard in vitro model for the study of drug-induced liver injury, toxicology and drug screening in humans is primary culture of freshly-isolated human hepatocytes. However, the use of primary human hepatocytes (PHH) is impeded by their limited availability, inter-donor differences, variable viability following isolation and rapid de-differentiation of the hepatocyte phenotype in culture. An induced pluripotent stem cell (iPSC)-based strategy allows for large-scale studies that would otherwise be impossible to perform on primary cell cultures or from biopsies and also enables studies on hepatocytes genetically susceptible to DILI as in vitro models with genotypic relevance for toxicology screening. This review summarises technical advances towards the generation of potentially clinically relevant human iPSCs. We discuss the derivation and characterisation of iPSC-derived hepatocyte-like cells (HLCs) and compare these to the gold standard: human biopsy-derived hepatocytes.
Generation of human iPSCs
Human iPSCs are pluripotent stem cells derived from somatic cells mediated by the ectopic expression of four transcription factors, namely OCT4, SOX2, KLF4 and c-MYC1 (Yamanaka factors) or OCT4, SOX2, NANOG and LIN282. The achievement of generating these iPSCs has meant that ethical as well as immune rejection issues associated with human embryonic stem cells3 can now be avoided (Figure 1).
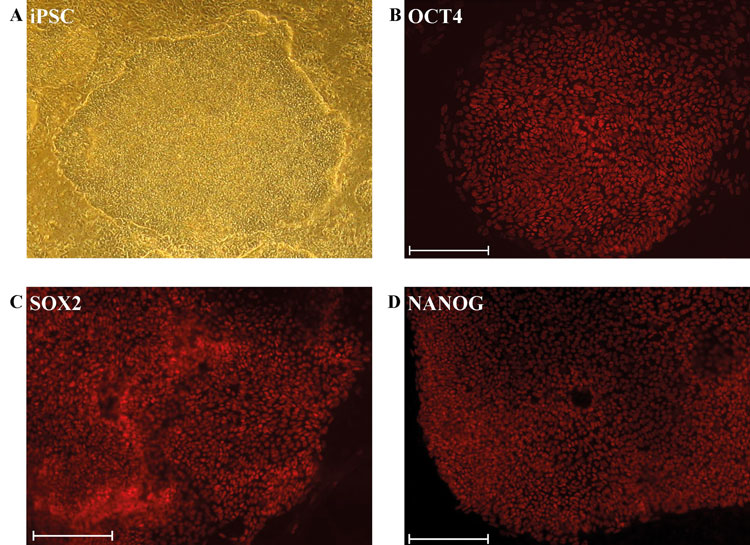
Figure 1: Derivation and characterisation of iPSCs
(A) Phase contrast image of an undifferentiated iPSC colony derived from dermal fibroblasts. Immunofluorescence-based detection of expression of the pluripotency-inducing proteins (B) OCT4/POU5F1 (C) SOX2 (D) NANOG. (Scale bar: 200μm)
Since the first generation of human iPSCs, retro- or lentiviral over-expression of the reprogramming factors have proven to be a robust and efficient way of inducing pluripotency in somatic cells. Efforts have been made to reduce or avoid integration of foreign DNA into the target cells’ genomes in order to minimise unpredictable effects of random integrations4-6.
Recently, Schlaeger et al.7conducted a robust comparison of non-integrating reprogramming protocols encompassing sendai virus, episomal and in vitro-derived mRNA-based delivery of the Yamanaka factors into human dermal fibroblasts. They concluded that the episomal-based reprogramming protocol is the most reliable for iPSC generation from fibroblast, CD34+ hematopoietic progenitor cells and peripheral blood mononuclear cells (PBMCs). Furthermore, the episomal-based protocol is well suited for clinical applications because it is integration-free and is based on plasmid DNA that can easily be generated using existing good manufacturing practice (cGMP)-compatible procedures.
Derivation and characterisations of HLCs
Hepatocyte-like cells (HLCs) derived from iPSCs are a valuable tool for disease modelling, drug testing and may have the potential to be surrogates for biopsy-derived primary hepatocytes. Over the years several protocols for the differentiation of human pluripotent stem cells into HLCs have been published8,9. Most protocols are based on three to four distinct steps, each induced by using a defined combination of basal medium and cytokines. First, cells are differentiated into definitive endoderm (DE) by using ACTIVIN A and optionally also WNT3A, bFGF and BMP410-14. In the second step, the DE cells are directed towards hepatic endoderm (HE), which is equivalent to a bipotential hepatoblast during hepatogenesis. Finally, maturation of HE cells into HLCs is induced by adding HGF, OSM, Dexamethasone and Insulin10-14. Depending on the protocol, HLCs can be derived after about 20 days in culture. The quality of the HLCs should be assessed by transcriptome11 proteome and also a host of biochemical assays, importantly the expression and secretion of ALBUMIN – a marker defining mature hepatocytes (Figure 2B). Functional activity tests comprise Cytochrome P450 activity, mostly measured as CYP3A4 activity, uptake and release of indocyanine dyes (transporter activity), glycogen storage and secretion of urea10-14. In most cases, HLCs show higher levels of CYP3A4 activity than the hepatocellular carcinoma line HepG2, but both cell types have lower activity than adult liver biopsy-derived primary hepatocytes (Figure 2D).
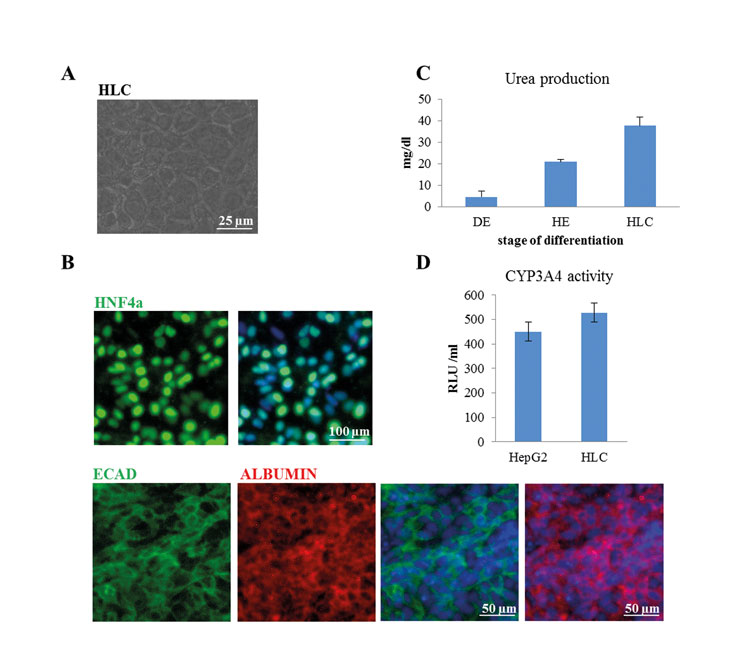
Figure 2: Characterisation of HLCs. Human iPSCs were differentiated into HLCs. (A) Morphology of HLCs was documented using light microscopy (Scale bar: 25µm). (B) HLCs express the hepatocyte-specific transcription factor HNF4α (Scale bar: 100µm) as well as ALBUMIN and E-CADHERIN (Scale bar: 50µm). (C) HLCs produce and secrete urea (DE-definitive endoderm, HE-hepatic endoderm, HLC-hepatocyte-like cells). (D) HLCs have cytochrome P450 enzyme CYP3A4 activity
Although HLCs described so far exhibit some characteristics of adult hepatocytes, they also retain some immature characteristics, such as relatively low level expression of Cytochrome P450 transcripts and the persistent expression of AFP, a marker of foetal rather than adult hepatocytes. However, several laboratories have made attempts to enhance the maturation of HLCs by optimising the extracellular matrix15, using 3D culture systems16, supplementation with small molecules17, co-culture with stromal-cells18 and micropatterned co-cultures19.
Drug metabolism and toxicology
Hepatocytes are central for characterising a candidate drug’s metabolism and drug potential. Various hepatocyte models have been developed for use in safety pharmacology and toxicology research to understand the mechanisms of DILI and to screen new chemical entities (NCEs) for their potential to cause adverse reactions. Freshly-isolated hepatocytes, cryopreserved hepatocytes, HepaRG and immortalised cancer cell lines (e.g., HepG2), liver tissue preparations (slices, microsomes and S9 fractions) and animal models broadly categorise the numerous hepatocyte models available for studies into the pathophysiology of DILI20,21. However, the utility and relevance of these models are limited.
The gold standard in vitro model for the study of DILI in humans is primary culture of freshly-isolated human hepatocytes from donated liver biopsies which invariably come from diseased and mostly liver cancer patients. Furthermore, the use of primary human hepatocytes (PHH) is impeded by their limited availability, inter-donor differences, variable viability following isolation and rapid dedifferentiation of the hepatocyte phenotype in culture, particularly in the loss of Cytochrome P450 (CYP) enzyme expression and activity. The limited life span and phenotypic instability also limits the utility of the PHH model to short-term studies only, and compromises their use in mechanistic studies of DILI which often occurs following prolonged exposure to drugs. Immortalised liver cancer cell lines such as HepG2 have been used to overcome these problems as they have an infinite life span and are readily available. However, they suffer from a deficit in metabolic activity (Figure 2D).
Most and perhaps all drugs promote their own metabolism by up-regulating the enzyme systems responsible for their degradation or activation (Phase I) and conjugation (Phase II) to biochemical forms that can be excreted in bile or urine. The majority of drugs that are metabolised by the liver through such detoxification pathways induce particular subsets of the Cytochrome P450 gene family22. The most ubiquitous of these, CYP3A4, is responsible for metabolising a large fraction of hepatotoxic drugs, and indeed CYP3A4 induction can be used as an index of exposure to them (Figure 2D). The expression of Cytochrome P450 family members has been demonstrated in iPSCs and ESCs derived hepatocyte-like cells10-14.
Figure 3 is a heatmap representation of genes encoding the core ADME enzymes based on the transcriptome data sets of iPSC- and ESC- derived HLCs10. These genes have been shown to be associated with drug metabolism and can be grouped into four distinct categories:
- Phase I-metabolism enzymes: CYP, DHRS and CBR;
- Phase II metabolism enzymes: GST, SULT, UGT, CHST and NAT, which are responsible for the modification of functional groups and the conjugation with endogenous moieties;
- transporters: SLC, ABC, MRP, which are responsible for the uptake and excretion of drugs in and out of cells; and
- modifiers: CAT, CDA, RXRA, PPARG, PPARD, SOD and SERPINA7, which can have dual functions, i.e., regulate the expression of other ADME genes or modulate the biochemistry of ADME enzymes.
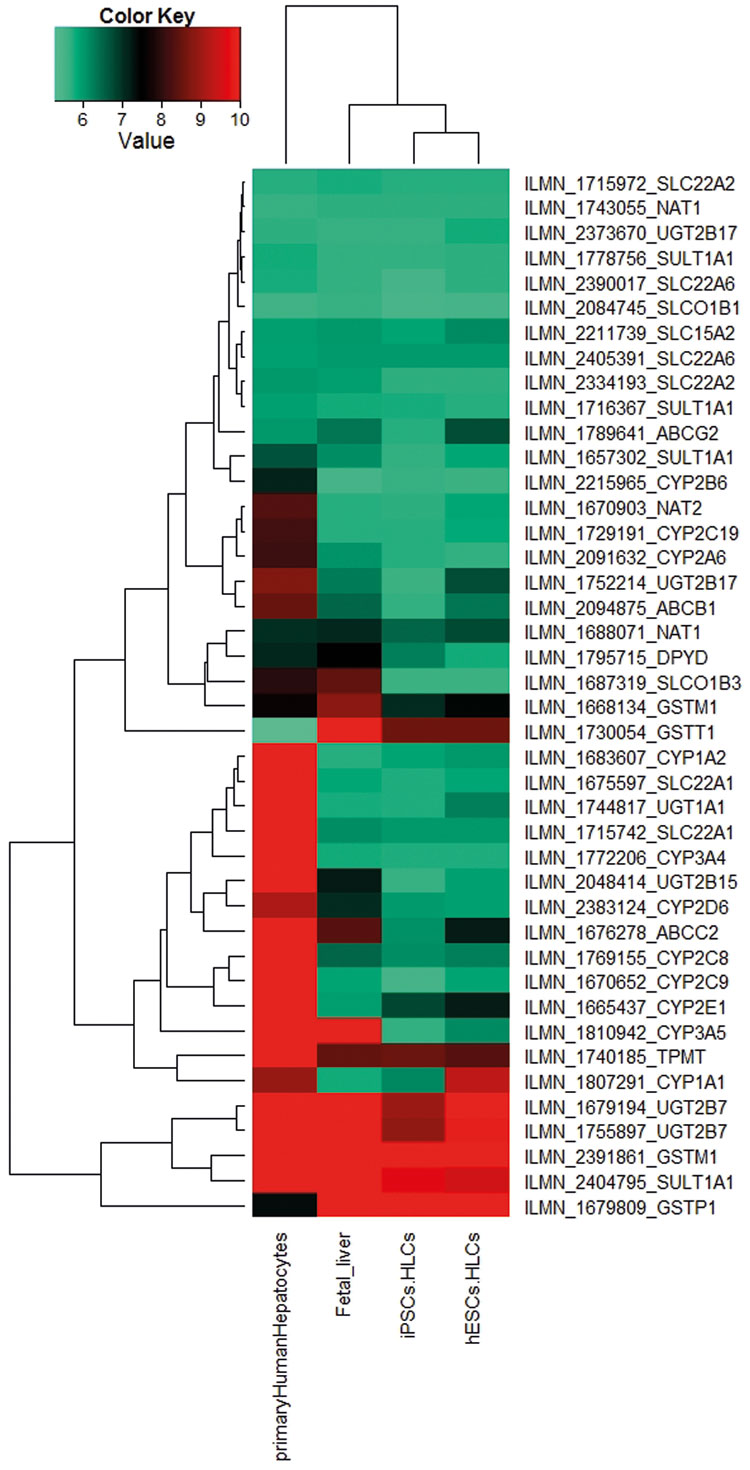
Figure 3: A heatmap-based representation of co-regulated expression of the core ADME genes (http://pharmaadme.org/joomla/index.php?option=com_content&task=view&id=14&Itemid=29) in adult human liver biopsy-derived primary hepatocytes, foetal liver, HLCs derived from foetal foreskin iPSCs (iPSCs.HLCs) and the human embryonic stem cell: H 1(hESCs.HLCs)10. The expression levels of these genes are higher in the adult primary hepatocytes
Impact on the 3Rs- Replacement, Reduction and Refinement
At present, testing in whole animals is still a major way to assess toxicity of drugs and other xenobiotics for humans, and to date pharmaceutical drugs have been validated by quantitative risk assessment determined by animal experimentation, assessing both local tissue-specific and whole organism systemic toxicity. These toxicology screens require the use of significant numbers of animals. Indeed, the testing of chemicals required under the EU Registration, Evaluation, Authorisation and Restriction of Chemicals (REACH) regulations has been estimated to require up to 54 million additional animals, therefore necessitating the development of alternative testing strategies
The implementation of iPSC-derived HLCs into toxicology screens would substantially reduce animal experiments in the future.
Perspectives
Pharmaceutical companies spend substantial funds on screening for metabolic properties early in drug discovery processes. The implementation of human iPSCs will significantly simplify this procedure as unpredicted human metabolism is one of the main causes of the withdrawal of potential new drugs from pharmaceutical projects. Principally, any cell type existing today does not copy the complexity and function of the liver. The human models available to date are utilising cancer cell lines or primary cells isolated from liver biopsies, and unfortunately, these two cell types possess significant limitation. Primary human hepatocytes are currently used as the gold standard in drug metabolism studies even though they lose functional properties when maintained in vitro.
In general, there is an urgent requirement for in vitro models of healthy and disease-specific hepatocytes. Hepatocytes and cardiomyocytes are the most susceptible to drug-induced damage (toxicity). It is very difficult to precisely predict hepatotoxic and cardiotoxic properties of new compounds in humans and in many cases toxicity is observed only in the late phases of clinical trials, due to the species-specific differences and extensive use of animal models. Although significant progress has been made in differentiating iPSCs into hepatocytes (HLCs), however, the efficient generation of a pure and mature cell population has not yet been accomplished. Undoubtedly, the standardisation of the production of functional human HLCs is needed. Only then will iPSC-derived hepatocytes significantly improve in vitro metabolism studies and toxicity trials.
Acknowledgements
James Adjaye acknowledges support from the medical faculty of the Heinrich Heine University- Düsseldorf, Germany.
Disclosure statement: The authors of this manuscript have nothing to declare.
References
- Takahashi K, Tanabe K, Ohnuki M, Narita M, Ichisaka T, Tomoda K, Yamanaka S (2007) Induction of pluripotent stem cells from adult human fibroblasts by defined factors. Cell 131:861-872.
- Yu J, Vodyanik MA, Smuga-Otto K, Antosiewicz-Bourget J, Frane JL, Tian S, Nie J, Jonsdottir GA, Ruotti V, Stewart Ret al (2007) Induced pluripotent stem cell lines derived from human somatic cells. Science 318:1917-1920.
- Thomson JA, Itskovitz-Eldor J, Shapiro SS, Waknitz MA, Swiergiel JJ, Marshall VS, Jones JM (1998) Embryonic stem cell lines derived from human blastocysts. Science 282:1145-1147
- Drews K, Jozefczuk J, Prigione A, Adjaye J.(2012). Human induced pluripotent stem cells–from mechanisms to clinical applications. J Mol Med (Berl) 90(7):735-45.
- Tavernier G, Mlody B, Demeester J, Adjaye J, De Smedt SC.(2013).Current methods for inducing pluripotency in somatic cells.Adv Mater. 25(20):2765-71.
- Katharina Drews and James Adjaye (2013). The promise held by induced pluripotent stem cells for research and regenerative medicine. European Pharmaceutical Review. Vol.18,Issue 2:21-25.
- Schlaeger TM, Daheron L, Brickler TR; et al. (2015). A comparison of non-integrating reprogramming methods. Nat Biotechnol. 33(1):58-63.
- Gerbal-Chaloin S, Funakoshi N, Caillaud A, Gondeau C, Champon B, Si-Tayeb K. (2014). Human induced pluripotent stem cells in hepatology: beyond the proof of concept. Am J Pathol;184:332-347.
- Dagmara Szkolnicka, wenli Zhou,Balta Lucendo-Villarin and David C. Hay (2013). Pluripotent stem cell-derived hepatocytes: Potential and challenges in pharmacology. Annu.Rev.Pharmacol. Toxicol. 53:147-59.
- Hay DC, Zhao D, Ross A et al. (2008). Highly efficient differentiation of hESCs to functional hepatic endoderm requires ActivinA and Wnt3a signaling. PNAS: 105(34):12301-6
- Jozefczuk J, Prigione A, Chavez L, Adjaye J. (2011). Comparative analysis of human embryonic stem cell and induced pluripotent stem cell-derived hepatocyte-like cells reveals current drawbacks and possible strategies for improved differentiation. Stem Cells Dev;20:1259-1275.
- Hannan NR, Segeritz CP, Touboul T, Vallier L.( 2013). Production of hepatocyte-like cells from human pluripotent stem cells. Nat Protoc;8:430-437.
- Loh KM, Ang LT, Zhang J, et al. (2014). Efficient endoderm induction from human pluripotent stem cells by logically directing signals controlling lineage bifurcations. Cell Stem Cell;14:237-252.
- Takayama K, Morisaki Y, Kuno Set al. (2014). Prediction of inter-individual differences in hepatic functions and drug sensitivity by using human iPS-derived hepatocytes. Proc Natl Acad Sci U S A 111: 16772-16777.
- Berger DR, Ware BR, Davidson MD et al. (2014). Enhancing the functional maturity of iPSC-derived human hepatocytes via controlled presentation of cell-cell interactions in vitro. Hepatology. doi: 10.1002/hep.27621.
- Gieseck RL, Hannan NR, Bort R, et al. (2014). Maturation of induced pluripotent stem cell derived hepatocytes by 3D-culture PLoS One;9:e86372.
- Shan J, Schwartz RE, Ross NT et al. ( 2013). Identification of small molecules for human hepatocyte expansion and iPS differentiation. Nat Chem Biol 2013;9:514-520.
- Takebe T, Sekine K, Enomura M et al. (2013).Vascularized and functional human liver from an iPSC-derived organ bud transplant. Nature;499:481-484.
- Brenton R. Ware, Dustin R. Berger and Salman R. Khetani (2015). Prediction of drug-induced liver injury in micropatterned co-cultures containing iPSC-derived human hepatocytes. Toxicol. Sci. doi: 10.1093/toxsci/kfv048
- Olson, H., Betton, G., Robinson, D. et al. (2000). Concordance of the toxicity of pharmaceuticals in human and in animals. Regulatory Toxicology and Pharmacology 32, 56-67.
- Ramaiahgari SC, den Braver MW, Herpers B et al.(2014). A 3D in vitro model of differentiated HepG2 cell spheroids with improved liver-like properties for repeated dose high-throughput toxicity studies. Arch Toxicol. 88(5):1083-95.
- Gerets HHJ, Tilman K, Gerin B et al. (2012). Characterization of primary human hepatocytes, HepG2 cells, and HepaRG cells at the mRNA level and CYP activity in response to inducers and their predictivity for the detection of human hepatotoxins. Cell biology and toxicology. 28, Issue 2, pp 69-87.
Biographies
Professor Dr. James Affram Adjaye has a BSc (Honours) degree in Biochemistry from University College of Cardiff, Wales, and an MSc in Biochemistry from the University of Sussex, Brighton. He has a PhD in Biochemistry from King’s College- London. Professor Adjaye was head of the Molecular Embryology and Aging group at the Max Planck Institute for Molecular Genetics, Berlin, Germany. He is now Director of the Institute for Stem Cell Research and Regenerative Medicine within the Faculty of Medicine at the Heinrich-Heine-University, Düsseldorf, Germany. He is involved in systems biology-based projects both at the national and international level where iPSCs are used to model Alzheimer’s Disease and Non-Alcoholic Fatty Liver Disease (NAFLD).
Dr. Nina Graffmann has a BSc and a MSc degree in Biochemistry from Heinrich-Heine University, Düsseldorf. She obtained her PhD in Düsseldorf investigating epigenetic changes during ageing. She now works as a Postdoctoral Fellow at the Institute for Stem Cell Research and Regenerative Medicine at Heinrich-Heine-University Düsseldorf and investigates Non-Alcoholic Fatty Liver Disease (NAFLD) using patient iPSC-derived hepatocyte-like cells as an in vitro model.
Mr Wasco Wruck has a diploma in computer science and a diploma in architecture from Technische Univerität Berlin, Germany. He worked as a software developer with Siemens AG and BULL AG before joining the Max-Planck-Institute for Molecular Genetics in Berlin. There he worked as a Bioinformatician on microarray-based image analysis, application development and to evaluate various types of high-throughput genomics experiments. He then worked on a European Systems biology research project at Charité Universitätsmedizin Berlin and was elected chair of the ERASysBio+ initiative’s data management group. He currently works at the Institute for Stem Cell Research and Regenerative Medicine at Heinrich-Heine-University Düsseldorf.
Miss Peggy Matz has a BSc in Biology from the Free University Berlin, Germany. Then she obtained a MSs in Molecular Life Science from Humboldt-University of Berlin, Germany. She is currently in the process of finishing her PhD at the Institute for Stem Cell Research and Regenerative Medicine within the Faculty of Medicine at the Heinrich-Heine-University, Düsseldorf, Germany. She is working on human iPSC generation and derivation of hepatocytes.
Related topics
Drug Discovery, Hepatocytes, Stem Cells
Related conditions
Liver disease
Related organisations
Institute for Stem Cell Research and Regenerative Medicine
Related people
Nina Graffmann, Peggy Matz, Prof Dr James Adjaye, Wasco Wruck