Innovations for next-generation antibody-drug conjugates
Posted: 3 September 2020 | Dr Amrik Basran (Avacta), Dr Matt Vincent (Avacta) | No comments yet
Dr Amrik Basran and Dr Matt Vincent discuss various developments for antibody-drug conjugates to treat cancer.
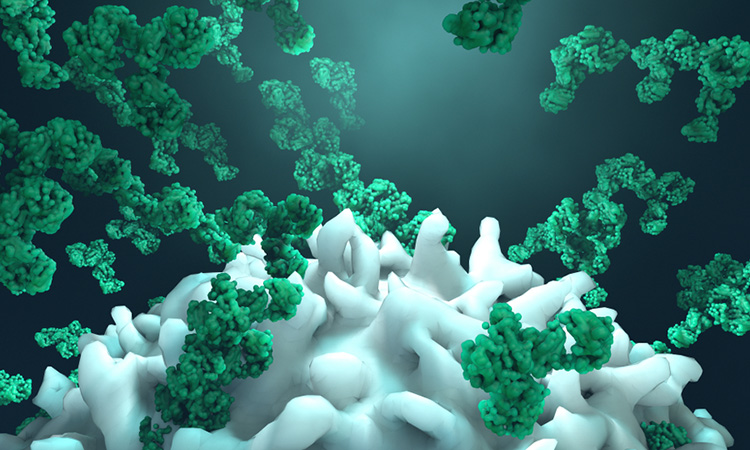
Antibody drug conjugates (ADCs) are a class of biopharmaceutical designed for the treatment of cancer, comprising an antibody that specifically targets a tumour antigen and a cytotoxic compound, connected via a chemical linker. ADCs are intended to allow the specific targeting of the cytotoxic compound to kill cancer cells, while having a limited effect on healthy tissue.
In practice however, ADCs developed over the past 40 years have seen mixed levels of success and have proved especially problematic for the treatment of solid tumours. In more recent times, we have seen some major advances in the development process, enabling the selection of the right tumour targets with the most appropriate toxic agents and the ability to design ADCs to kill nearby cancer cells that had previously evaded destruction. This has led to a total of eight ADCs receiving market approval for oncotherapy since 2011.1
ADCs – a multicomponent drug design challenge
The design of ADC therapeutics is highly complex, so an in-depth understanding of several research areas to successfully develop these molecules is required. These include the nature of the tumour antigen biology, the targeting platform used to deliver the drug (eg, antibodies), the conjugation chemistry used to attach the linker and payload to the protein, linker chemistries and the types of cytotoxic payloads used; all of which still present challenges that will affect tissue specificity, tumour penetration, payload release, efficacy and off-target toxicity.
Tumour antigens and targeting platforms
The selection of the tumour target for ADC development needs to meet certain criteria. For example, the target antigen needs to be expressed at high levels on the tumour cells but at low levels on healthy tissue to ensure a high level of selective cell killing, otherwise unacceptable levels of toxicity may be observed. The ADC/target antigen complex needs to be rapidly internalised in order to efficiently release the cytotoxic payload.2 The tendency for the antigen to be shed from the cell surface can also impact the efficacy of the ADC and is dependent on the ratio of the target on the cell surface and in circulation. For example, it may be beneficial to have high levels of circulating target antigen if it is highly expressed on the target cell.3
The large size, long serum half-life and high affinity of antibodies can also be an issue when trying to achieve tumour penetration. High affinity interactions with the target may cause the ADC to remain bound at the periphery of the tumour rather than penetrating deep within the tissue, especially with large tumours.4,5
Novel platforms based on smaller binding proteins, often referred to as antibody mimetics, eg, domain antibodies, DARPins®, nanobodies and Affimer™ proteins, hold potential benefits for tumour penetration. This is due to their smaller size (ranging from 14-20kDa depending on the platform) and a greater scope to modulate the pharmacokinetic (PK) profile in vivo for greater tumour/serum ratios for improved cell killing compared to antibodies.6 At ~2-3kDa in size, Bicycle Therapeutics’ conjugates have much better tissue penetration and lower systemic exposure due to their rapid clearance compared to antibody-based ADCs. Bicycle is also targeting metalloproteases in the tumour microenvironment for improved selectivity. Ablynx and Crescendo Therapeutics have additionally generated single domain antibody ADCs.
Linker design and payloads
A variety of linker chemistries have been used in ADCs, most requiring internalisation of the ADC for release of the drug moiety. As well as attaching the cytotoxic payload to the antibody, the linker design plays a critical role in the safety and efficacy of the final drug. The biophysical properties of the linker, such as solubility, must also be carefully designed to prevent any detrimental impact on the PK and pharmacodynamics (PD) of the final drug.7 The linker needs to be stable and remain conjugated to the protein in circulation to minimise the early release of the cytotoxin, thus minimising systemic toxicity, but labile once the ADC is within the tumour or cancer cell to release the payload.
The classic approach with ADCs has been to target tumour antigens”
The majority of linkers in use in ADCs are based on chemical motifs, including disulphide-containing linkers, hydrazones, peptides (cleavable) or thioethers (non-cleavable). Non-cleavable linkers require the entire drug complex to enter the cell and then be degraded in the lysosome to release the payload, whereas cleavable linkers are designed to break preferentially in the tumour cell under certain conditions. This could be from a change of pH or difference in reduction potential between the intracellular versus extracellular compartment. This allows the efficient release of the cytotoxic payload to either kill the cell or diffuse away from the targeted cell with the potential to be taken up by nearby cells and destroy them as well (known as the ‘bystander effect’). Another approach is to use a linker that can be cleaved by the action of enzymes such as extracellular proteases (matrix metalloproteases or FAP-α) or intracellular enzymes such as lysosomal cathepsin.
Early ADCs used clinically proven chemotherapeutics such as doxorubicin and methotrexate as the payload, but it became apparent that due to the low potency of the payloads, not enough toxin could be delivered by the ADC to the tumour to demonstrate efficacy.8 Higher potency molecules have been shown to have less systemic toxicity and improved therapeutic index. The majority of payloads now being developed fall broadly into two categories of DNA damaging compounds (eg, Calicheamicin and pyrrolobenzodiazepines) or tublin polymerisation inhibitors (eg, auristatins and maytansinoids).
Conjugation chemistry
Early ADCs relied on random NHS chemistry to attached the linker-toxin payload to the antibody via lysine residues. Due to the numerous lysine residues available for conjugation within the antibody, this usually resulted in a wide heterogenous population of products being generated, with differing drug/antibody ratios (DAR). This makes reproducibility for manufacturing challenging, as well as potentially affecting the PK, PD and therapeutic index of the final product. Site specific conjugation using, for example, maleimide chemistry and cysteine residues that are either naturally occurring or engineered in, have significantly improved the ability to control the DAR and reduce product variability. Advances in maleimide conjugation chemistry been developed by several companies, including Abzena with their proprietary CyPEG™ chemistry and Iksuda Therapeutics’ vinyl pyridine-based PermaLink® technology. Both approaches have improved conjugation stability compared to maleimide chemistry, which can undergo retro-Michael reaction allowing ring-opening of the maleimide and premature deconjugation from the ADC.9
Incremental technology improvements
ADCs are large, complex, multicomponent drugs”
As our understanding of how each component of the ADC can be optimised to produce a drug targeting delivery platform has improved, this has led to a range of new approaches being developed that could enhance the way both liquid and solid tumours are treated, to address some of the failings we see currently with ADCs. The limitations and failures of some ADCs has been attributed to unmanageable toxicity and poor efficacy due to low drug internalisation rates, low levels of target antigen expression and off-target toxicities due to premature payload release. This has led scientists to develop novel approaches to address these challenges, outlined below.
Bispecific targeting ADCs
Protein engineering approaches have been used in order to engineer two different targeting sites in the same molecule, generating bispecific molecules that can target more than one tumour antigen or two different epitopes on the same antigen (biparatopic), improving their mechanism of action (MoA) and tumour targeting selectivity. However, the linker chemistry here still requires the bispecific to be internalised with fairly rapid kinetics and the associated drug moiety is directly and principally toxic to the tumour cell.
In theory, bispecific ADCs should offer patient benefit in terms of improved MoA and efficacy and be cheaper to manufacture compared to manufacturing two separate ADCs for co-administration. But the reality is that the industry has struggled to develop effective bispecifics over the past 10 years and currently only a couple have been approved and taken to market. The problem lies partly in the stability of the molecules, which can cause issues in manufacturing at scale for clinical studies, but also in the understanding of the underlying target biology from pre-clinical models and how it will translate to the disease in humans. For smaller biotech companies target selection and validation is a particular challenge, as it is an expensive and time intensive process. The knowledge and data about which target combinations may be successful has thus far mainly been researched in academic institutions or in collaboration with larger pharma companies.
Combining ADCs with immunotherapy
The classic approach with ADCs has been to target tumour antigens, but as our knowledge of the immune system and the role of some of the key regulators such as immune checkpoints (ICP) has increased, the landscape for potential treatments in oncology is also changing. We are now starting to see research into the potential of combining cytotoxic chemotherapeutics (including ADCs) that modulate the innate immune system, with antibodies (or antibody mimetics) that can modulate the adaptive immune system (eg, via PD-L1/PD-1 blockade), to generate a tumour-specific immunological memory and more durable response in a wider group of patients.
Combining chemotherapies/ADCs with ICP antagonists is highly challenging and complex due to the nature of the human immune system, the heterogeneity that exists within tumour cell populations and the complex nature of the pre-clinical models available for testing and how well they represent the actual disease in a human. Consequently, researchers are beginning to look into the possibility of generating therapies that use the ICP as both a tumour target but also to modulate the immune system within the tumour, ie, ICP antagonist-drug conjugates that are able to activate both the innate and adaptive immune systems.10
The classical approach used for ADCs requires internalisation to enable the toxic payload to be cleaved by intracellular proteases. Internalisation occurs slowly with certain ICPs, so the payload needs to be released extracellularly within the tumour. A level of extracellular selectivity can be achieved by using pH sensitive linkers, but a superior approach involves targeting proteases that are upregulated in the tumour stroma, such as FAP-α, to release the toxic payload from the linker FAP-α is expressed on, for example, cancer associated fibroblasts. As a tumour target it is not new – many groups are targeting molecules, such as interleukins, to the tumour using antibodies or antibody mimetics which bind to FAP.
Work carried out with Bill Bachovchin at Tufts Medical School has generated anti-PD-L1-Affimer drug conjugates that utilise a novel FAP-α cleavable linker to release the toxin. This capitalised on the knowledge that FAP-α is a post-proline cleaving enzyme that is upregulated in the tumour compared to healthy tissue. Similarly to classic ADCs, the drug moiety is inert in the conjugated form, but upon FAP-α cleavage in the tumour stroma, the drug is released in its most active form. In this case, the warhead is an i-DASH inhibitor that selectively kills macrophages causing activation of the innate immune system via pyroptosis. At the same time, the anti-PDL1 inhibitory protein modulates the immune system to allow the influx of other immune cells.
In a tumour-bearing mouse syngeneic model, this approach has shown both full tumour regression in several individuals and the generation of a tumour-specific immunological memory effect. Tumour targeting of ICP proteins can be achieved using antibodies or alternative scaffolds, such as Affimer proteins, DARPins, etc. Coupling these proteins to drug moieties which act not only on the tumour cells, but also on the stroma or immune cells, allows the targeting of different anti-cancer immune pathways and hopefully an improved clinical outcome for patients.
Conclusion
ADCs are intended to allow the specific targeting of the cytotoxic compound to kill cancer cells”
The academic, biotech and pharma sectors are capitalising on our rapidly accelerating knowledge of both how to develop complex ADCs and the wider understanding of tumour biology and the interaction with the immune system, with the ultimate aim of providing safer and more effective treatments for cancer patients. As ADCs are large, complex, multicomponent drugs, innovative solutions have been created to address the many technical challenges and generate new improved cancer therapies. Although ADCs have historically been beset with difficulty, these new advances are beginning to offer some solutions. Overcoming issues such as acute and chronic treatment-related toxicities will be crucial, as will easing the financial burden to healthcare providers of antibody-based immunotherapies. Targeting multiple therapeutic pathways and enabling synergy of the innate and adaptive immune responses may hold the key to the future of ADCs and immuno-oncology.
About the authors
Dr Amrik Basran is Chief Scientific Officer at Avacta and has over 14 years of experience in biotech and pharma, with a strong scientific background in protein biochemistry and engineering.
Dr Matt Vincent is Vice President of Therapeutics Business Development at Avacta. He has over 25 years of experience in multiple areas of biotechnology and life sciences.
References
- The Antibody Society. Antibody Therapeutics Approved or in Regulatory Review in the EU Or US. [Internet] [Cited 20 Aug 2020] Available from: https://www.antibodysociety.org/resources/approved-antibodies/
- Ericksoabout:blankn HK et al. Antibody-Maytansinoid Conjugates Are Activated in Targeted Cancer Cells by Lysosomal Degradation and Linker-Dependent Intracellular Processing. Cancer Res. 2006 66(8), p.4426–4433. doi: 10.1158/0008-5472.can-05-4489
- Pak Y, Zhang Y, Pastan I, Lee B. Antigen Shedding May Improve Efficiencies for Delivery of Antibody-Based Anticancer Agents in Solid Tumours. Integrated Systems and Technologies: Mathematical Oncology, Cancer Research. 2012 72(13): p. 3143-3152 https://cancerres.aacrjournals.org/content/72/13/3143.full-text.pdf
- Nessler I et al. Increased Tumor Penetration of Single-Domain Antibody–Drug Conjugates Improves In Vivo Efficacy in Prostate Cancer Models. Cancer Research. 2020 80(6); p. 1268-78 https://cancerres.aacrjournals.org/content/80/6/1268
- Tang H et al. The Analysis of Key Factors Related to ADCs Structural Design. Front. Pharmacol. 2019 10:373. https://doi.org/10.3389/fphar.2019.00373
- Tolcher, A.W et al. Randomized phase ii study of br96-doxorubicin conjugate in patients with metastatic breast cancer. J. Clin. Oncol. 1999, 17, p.478–484
- Frigerio M and Kyle AF, The Chemical Design and Synthesis of Linkers Used in Antibody Drug Conjugates, Curr. Top. Med. Chem. 2017 17(32): p.3393-3424
- Sau S et al. PDL-1 Antibody Drug Conjugate for Selective Chemo-Guided Immune Modulation of Cancer. Cancers 2019, 11, 232
Related topics
Antibodies, Drug Development, Enzymes, Protein, Proteomics, Research & Development
Related conditions
Cancer
Related organisations
Ablynx, Abzena, Bicycle Therapeutics, Crescendo Therapeutics, Iksuda Therapeutics, Tufts Medical School
Related people
Bill Bachovchin