Novel systems for realising the promise of DNA vaccines and enabling the next generation of genetic medicines
Posted: 5 April 2022 | Dr John Lewis (Entos Pharmaceuticals) | 1 comment
Dr John Lewis, CEO of Entos Pharmaceuticals, outlines how an innovative proteolipid platform could enable a new class of DNA-based vaccines in this article.
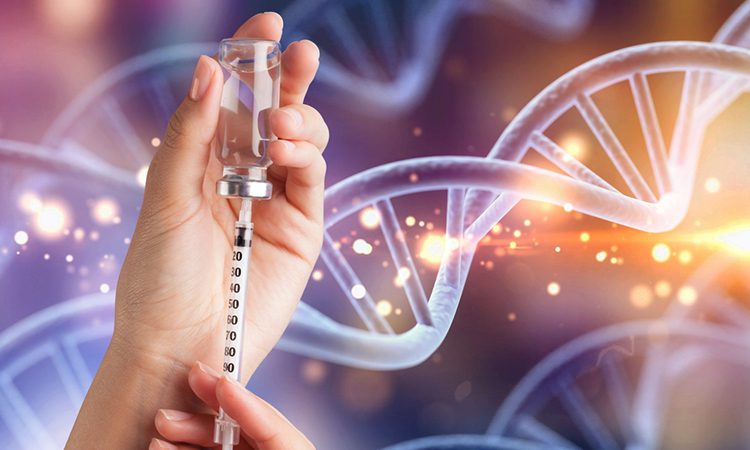
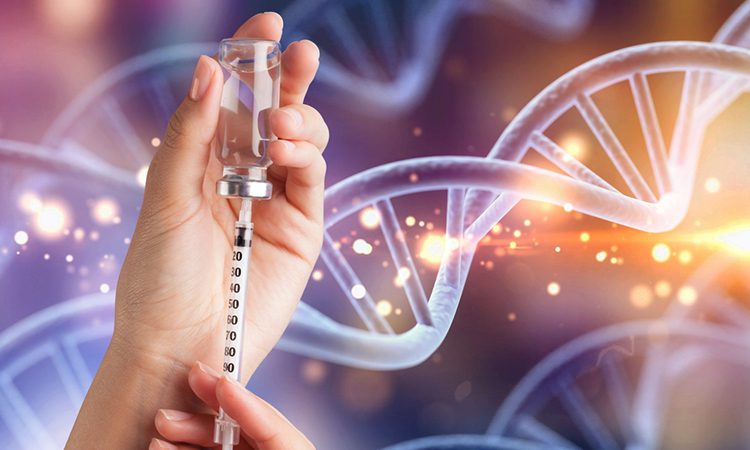
Enjoying this article by Dr John Lewis? Then join him as well as Sijme Zeilemaker, CEO of Immunetune and Thomas Tillet, President & Co-Founder of MBF Therapeutics, at our Development of Next-Generation Vaccines Online Summit this June. They will be speaking during the panel discussion ‘The broad potential of DNA vaccines’ which will be moderated by Dr Gary McLean, Professor at London Metropolitan University. During this session, they will discuss the advantages of delivering DNA that encodes for target antigens and why this strategy is a particularly promising vaccine platform.
The success of messenger RNA (mRNA) vaccines against SARS-CoV-2 has renewed interest in the broader development of nucleic acid-based vaccine technologies. Both mRNA and DNA vaccines deliver nucleic acid sequences that encode viral or tumour antigens designed to stimulate the production of neutralising antibodies and T cells. Despite these similarities, DNA-based vaccines offer multiple therapeutic and supply chain benefits compared with RNA-based vaccines.
DNA is an inherently more stable molecule and nucleic acid sequences delivered with DNA vaccines persist much longer”
mRNA is inherently unstable and mRNA vaccines, therefore, are limited by the rapid degradation of RNA which results in the relatively brief expression of encoded antigens. This transient expression may account for the short-term duration of protection observed with COVID-19 mRNA vaccines.
mRNA-based vaccines also have practical limitations resulting from their requirement for cold or ultra-cold chain handling, which can complicate supply chain logistics. While meeting these requirements may be feasible in areas with well-developed infrastructure, they may not be practical, or cost-effective in low- or middle-income countries1 or in rural or less developed areas. These challenges reduce the potential utility of mRNA vaccines and contribute to global inequities in healthcare.
In contrast, DNA is an inherently more stable molecule and nucleic acid sequences delivered with DNA vaccines persist much longer than those delivered with mRNA vaccines. This leads to a prolonged period of antigen expression during which the immune system can be trained to recognise and respond to target antigens. Prolonged antigen expression may produce more durable immune protection by stimulating more robust T cell-mediated immunity, including CD8+ T cells.
In addition, DNA vaccines have an amplifying effect where multiple mRNA molecules encoding the target antigen are generated from each DNA molecule, leading to significantly higher antigen expression and amplifying immune stimulation beyond what can be achieved with RNA vaccines. This may also stimulate more potent immune responses that could enable single-dose vaccination strategies or single boosters following mRNA vaccination.
Beyond their improved pharmacokinetics compared with mRNA vaccines, DNA vaccines can be engineered to encode multiple epitopes from a single antigen or epitopes from multiple antigens.2 This would support the development of vaccines that stimulate a broader and more diverse immune response for better protection against viral variants and enable multi-valent vaccines that could provide protection against multiple pathogens, such as a single vaccine that could protect against all coronaviruses. Moreover, DNA vaccines can also be engineered to deliver genetic adjuvants with the potential to stimulate enhanced immune responses.3
Finally, DNA vaccines are highly stable and can be shipped and stored under standard refrigeration conditions, simplifying supply-chain logistics and enabling their use in resource-constrained settings.
Several approaches have been evaluated for their potential to enhance transduction efficiency. Adenovirus- and adeno-associated virus (AAV)-based delivery systems more efficiently deliver DNA to the nucleus than naked DNA, but antiviral immune responses may lead to adverse events and also make re-dosing not possible.6,7 Electroporation, which uses brief electrical pulses to increase cell membrane permeability, can effectively deliver DNA to the nucleus but is painful and requires special and costly equipment.2 Additionally, although electroporation may be more efficient than naked DNA, it still requires milligram doses, making it impractical to implement in routine clinical or public health practice.8,9 The use of lipid nanoparticles (LNPs) allows for endosomal uptake of DNA, similar to RNA, but can lead to significant toxicity.10,11
Realising the promise of DNA vaccines requires novel delivery systems that combine the best aspects of LNP and viral delivery systems to deliver DNA efficiently and safely to the nucleus.
Such systems can be achieved by embedding viral fusion proteins (VFP) in LNPs to enable more effective delivery to the nucleus without the immune response-related challenges of viral vectors. Initial efforts to develop a VFP/LNP delivery system utilised extracellular vesicles (EVs) that incorporated VFP derived from an enveloped virus. However, this VFP is a large and highly immunogenic protein12 that has the potential to induce adverse immune responses and may limit the ability to re-dose.
DNA vaccines can be engineered to encode multiple epitopes from a single antigen or epitopes from multiple antigens”
More recently, a new approach was enabled by the discovery of a family of non-enveloped reoviruses that express novel VFPs known as fusion-associated small transmembrane (FAST) proteins. In contrast to enveloped virus VFPs, FAST proteins are small and show minimal immunogenicity.13 Recombinant FAST proteins can be cost-effectively produced at scale using standard biomanufacturing approaches in baculovirus or Chinese hamster ovary (CHO) cell systems. A recombinant chimeric FAST protein engineered for optimal efficacy was combined with well-tolerated neutral lipids to formulate the Fusogenix Proteolipid Vehicle (PLV) platform. Fusogenix PLVs fuse with the host cell to deliver nucleic acid payloads directly inside the cell, bypassing the endosomal pathway to promote efficient intracellular DNA delivery13-15 and enabling a new class of DNA-based vaccines and therapeutics.
The COVID-19 DNA vaccine candidate currently being evaluated in Phase II clinical trials has demonstrated proof-of-concept for the novel Fusogenix PLV platform. This candidate vaccine is engineered to encode SARS-CoV-2 spike protein as well as sequences encoding genetic adjuvants.16, 17
The Fusogenix PLV platform demonstrates efficient intracellular delivery of a wide range of nucleic acid payloads including DNA, mRNA, regulatory RNA, gene editing (CRISPR) molecules and multiplexed payloads, with a favourable, low-toxicity profile.14
Fusogenix PLVs are currently being developed and evaluated in diverse indications including oncology therapeutics, DNA vaccines, age-related diseases, genetic diseases and neurological disorders.18 Initiation of additional clinical programmes is expected to add to the growing body of data supporting the potential of the Fusogenix PLV platform to transform the genetic medicine landscape and enable new therapeutic and vaccine approaches that can address unmet needs and improve patient outcomes.
About the author
Dr John Lewis is the Founder and Chief Executive Officer of Entos Pharmaceuticals, which develops next generation nucleic acid-based therapies using the proprietary Fusogenix proteo-lipid vehicle (PLV) drug delivery system. He is also a Professor at the University of Alberta, Canada.
References
- Pardi, N., Hogan, M.J., Porter, F.W., and Weissman, D. (2018). mRNA vaccines — a new era in vaccinology. Nat Rev Drug Discov 17.
- Jahanafrooz, Z., Baradaran, B., Mosafer, J., Hashemzaei, M., Rezaei, T., Mokhtarzadeh, A., and Hamblin, M.R. (2020). Comparison of DNA and mRNA vaccines against cancer. Drug Discov Today 25, 552-560.
- Suschak, J.J., Dupuy, L.C., Shoemaker, C.J., Six, C., Kwilas, S.A., Spik, K.W., Williams, J.A., and Schmaljohn, C.S. (2020). Nanoplasmid Vectors Co-expressing Innate Immune Agonists Enhance DNA Vaccines for Venezuelan Equine Encephalitis Virus and Ebola Virus. Molecular Therapy – Methods & Clinical Development 17, 810-821.
- Ulmer J.B., Donnelly J.J., Parker S.E., Rhodes G.H., Felgner P.L., Dwarki V.J., Gromkowski S.H., Deck R.R., DeWitt C.M., Friedman A. Heterologous protection against influenza by injection of DNA encoding a viral protein. Science. 1993;259:1745–1749.
- Fynan E.F., Webster R.G., Fuller D.H., Haynes J.R., Santoro J.C., Robinson H.L. DNA vaccines: Protective immunizations by parenteral, mucosal, and gene‐gun inoculations. Proc. Natl. Acad. Sci. USA. 1993;90:11478–11482
- Wang, D., Tai, P.W.L., and Gao, G. (2019). Adeno-associated virus vector as a platform for gene therapy delivery. Nature reviews Drug discovery 18, 358-378.
- Thomas, C.E., Ehrhardt, A., and Kay, M.A. (2003). Progress and problems with the use of viral vectors for gene therapy. Nature Reviews Genetics 4, 346-358.
- Smith, T.R.F., Patel, A., Ramos, S., Elwood, D., Zhu, X., Yan, J., Gary, E.N., Walker, S.N., Schultheis, K., Purwar, M., et al. (2020). Immunogenicity of a DNA vaccine candidate for COVID-19. Nature Communications 11, 2601.
- Yu, J., Tostanoski, L.H., Peter, L., Mercado, N.B., McMahan, K., Mahrokhian, S.H., Nkolola, J.P., Liu, J., Li, Z., Chandrashekar, A., et al. (2020). DNA vaccine protection against SARS-CoV-2 in rhesus macaques. Science 369, 806-811.
- Zhang, J.-S., Liu, F., and Huang, L. (2005). Implications of pharmacokinetic behavior of lipoplex for its inflammatory toxicity. Advanced Drug Delivery Reviews 57, 689-698.
- Zolnik, B.S., González-Fernández, A., Sadrieh, N., and Dobrovolskaia, M.A. (2010). Nanoparticles and the immune system. Endocrinology 151, 458-465.
- White, J.M., Delos, S.E., Brecher, M., and Schornberg, K. (2008). Structures and mechanisms of viral membrane fusion proteins: multiple variations on a common theme. Crit Rev Biochem Mol Biol 43, 189-219.
- Ciechonska, M., and Duncan, R. (2014). Reovirus FAST proteins: virus-encoded cellular fusogens. Trends in microbiology 22, 715-724.
- Brown, D., Wee, P., Bhandari, P., Parmar, M., Vega, H., Grin, L., Sosnowski, D., Hejazi, M., Ablack, J., Clancy, E.K., et al. (2022). Safe and effective delivery of nucleic acids using proteolipid vehicles formulated with fusion-associated small transmembrane proteins (In Submission).
- Duncan, R. (2019). Fusogenic Reoviruses and Their Fusion-Associated Small Transmembrane (FAST) Proteins. Annual review of virology 6, 341-363.
- Raturi, A., Ablack, J., Wee, P., Bhanduri, P., Parmar, M., Brown, D., Hejazi, M., McMullen, N., Grin, L., Vega, H., et al. (2022). Immunogenicity of SARS-CoV-2 DNA Vaccine Candidates Formulated with the Fusion-Associated Small Transmembrane Protein Proteolipid Vehicle Delivery Platform (In Submission).
- Cavalcanti, I.D.L., and Cajubá de Britto Lira Nogueira, M. (2020). Pharmaceutical nanotechnology: which products are been designed against COVID-19? Journal of nanoparticle research: an interdisciplinary forum for nanoscale science and technology 22, 276-276.
- Brown, D., Raturi, A, Bhandari, P., Sosnowski, D., Grin, L., Wee, P., Vega, H, Gyoba, J, Hejazi, M., et al. Selective ablation of solid tumors using a p53-targeted FAST-LNP gene therapy [abstract]. In: Proceedings of the Annual Meeting of the American Association for Cancer Research 2020; 2020 Apr 27-28 and Jun 22-24. Philadelphia (PA): AACR; Cancer Res 2020;80(16 Suppl):Abstract nr 4069.
Related topics
DNA, Drug Delivery, Immunology, Lipidomics, Vaccine
Related conditions
Covid-19
John Sanford and I first report immunizing with DNA (not referenced). We used the gene gun. If DNA is delivered to cytoplasm not as a bollus, it is directly transported to nucleus. There is no need for special transport. We also noted the “simian barrier” of the peculiar problem of Gene vaccines in primates and humans. There is probably a simple solution to this problem.