Human neuronal cells: possibilities in drug safety testing
Posted: 26 September 2023 | Dr Kimberly Rockley (ApconiX) | No comments yet
Human derived induced pluripotent stem cells (hiPSCs) have revolutionised research and are increasingly used for toxicology screening and disease modelling. Early detection of neurotoxicity induced by potential new therapies is a major challenge, and hiPSC-neuronal cells may provide a solution. These cells demonstrate considerable promise for uncovering drug-induced perturbations to neuronal function such as seizure, […]
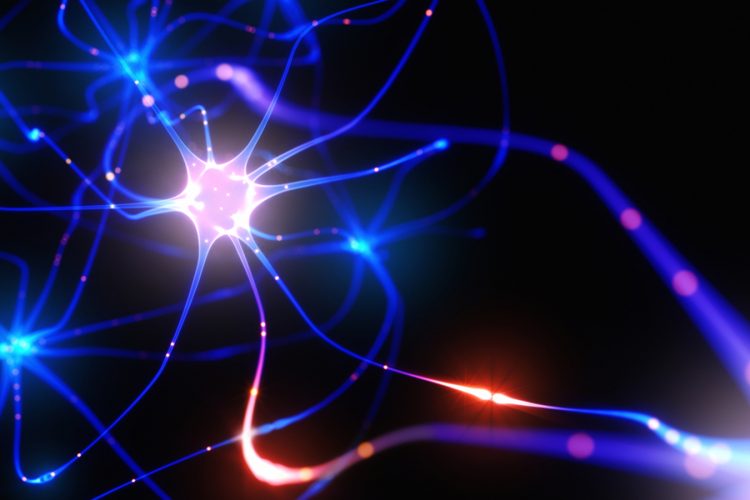
Human derived induced pluripotent stem cells (hiPSCs) have revolutionised research and are increasingly used for toxicology screening and disease modelling. Early detection of neurotoxicity induced by potential new therapies is a major challenge, and hiPSC-neuronal cells may provide a solution. These cells demonstrate considerable promise for uncovering drug-induced perturbations to neuronal function such as seizure, and their use extends further to sedation, anti-epileptic drug discovery and modelling of neurological diseases.
Within the field of drug safety hiPSC-cardiomyocytes are now routinely used for early identification of cardiac liabilities in drug discovery. This shift occurred following implementation of the Comprehensive in Vitro Proarrhythmia Assay (CiPA) initiative in 2013 and extensive testing of hiPSC-cardiomyocytes with known proarrhythmic drugs. The CiPA initiative aims to engineer an assay to assess the proarrhythmic potential of new drugs with improved specificity compared to current methods. CiPA focusses on the use of emerging new technologies and incorporates ventricular ion channel screening, in silico methods and hiPSC-cardiomyocyte analyses to form an integrated assessment of proarrhythmic risk.1-4
This approach saves time, money, and resources, and ultimately leads to safer medicines going forward to the clinic.
Another area in need of attention is central nervous system (CNS)-safety testing. CNS-related issues account for nearly a quarter of failures during clinical development, a phase where consequences are high in terms of resources and patient impact.5,6 The range of CNS-related toxicities encountered throughout drug development and post-market approval is vast, and includes abuse liability, suicidal ideation, emesis, sleep disorder and cognitive dysfunction to name a few.7,8 Modelling any of these endpoints in vitro would be especially challenging, however other toxicities that involve perturbations in electrical activity such as seizure may be amenable to earlier detection. Seizures and tremors occur frequently in preclinical drug development and were encountered by 67 percent and 65 percent respondents of a nervous system focussed industry survey respectively.7
The same industry survey highlights that the most frequently used methods for seizure liability assessment are observations/EEG in preclinical rodent and non-rodent studies and the rodent hippocampal slice assay. These models all share similar limitations concerning their low throughput, interspecies comparison, and cost. It is well known that there are differences between rodent and human brains,9 so a shift towards human-based models seems like a logical step in the right direction. Interestingly, stem cell derived neurons were used by only 15 percent respondents as part of their CNS safety assessments7
Is it time to act and increase this number whilst decreasing our reliance on animal studies with questionable translation?
The cardiovascular safety revamp was a great success story, perhaps we can improve seizure liability testing in the same way?
A seizure is characterised by periods of excessive neuronal firing and uncontrolled hyper-excitability.10 The use of microelectrode arrays (MEA) to monitor spontaneous electrical activity and drug responses in hiPSC-neuronal cultures stands out as a suitable method to identify seizure liability in vitro. This approach enables high-throughput non-invasive measurement of electrical activity from a network of heterogenous neuronal cells. This has great potential for predicting seizure liability of drug candidates as changes in neuronal firing can be measured in real-time with millisecond temporal resolution using specialised plates with electrodes. This allows for analysis of multiple parameters that illustrate the activity of neuronal networks.11
Any potential in vitro method for seizure liability testing needs to be reproducible and mimic the complexity of the human brain without being too technically demanding. The recent introduction of commercially available hiPSC-neuronal cells of multiple cell types may provide a solution to issues such as batch-to-batch variation and long, complicated differentiation processes. Commercial cells require a relatively short culture time and neurons can be mixed at desired ratios to mimic the human brain.
Normal activity in the brain relies on a balance between inhibitory neurotransmission and neuronal excitation, so our hiPSC-cultures need to contain the right neurons to achieve this balance. We found that a mixture of excitatory glutamatergic neurons (68 percent), inhibitory GABAergic neurons (17 percent) and supportive astrocytes (15 percent) performed well at detecting seizurogenicity of sixteen known seizurogenic compounds with various mechanisms of action. Most of these compounds behaved as expected and caused seizure-like behaviour, with one or two exceptions. These results are presented in table 1, alongside rodent hippocampal slice data, which are frequently used in projects where issues with seizure are anticipated.12,13 We found good alignment between the two models, providing further evidence of the benefit of our assay.
It is now appreciated that hiPSC-cardiomyocytes from different sources and vendors display different electrophysiologic phenotypes which may reflect different levels of maturation. These differences can affect pharmacologic responses and influence assay sensitivity and subsequent risk assessments.14 Similarly, we found that the ratio of cell types is a crucial consideration for detection of different mechanisms of seizurogenic aetiology. For example, in co-cultures containing fewer GABAergic neurons we found that picrotoxin and pilocarpine had no effect. This is presumably due to a lack of extra-synaptic GABA for picrotoxin, and a lack of expression of the relevant target, likely the M1 muscarinic receptor, for pilocarpine. Interestingly, 4-AP caused a dramatic decrease in activity in this culture. This may be due to differential expression of Kv channels – 4-AP is a highly potent inhibitor of Kv3.1 which is expressed on inhibitory GABAergic neurons, and less potently inhibits other Kv channels present on excitatory neurons.15,16
The trickiest group of compounds to nail down in our hiPSC neuronal co-culture were the GABA-A antagonists. We observed clear, expected changes with picrotoxin and bicuculline, but these changes are subtle compared to the changes observed in primary rodent cortical cultures.17 This could be due to differential expression and activity of GABA-B receptors across the cell models. GABA-B receptors are GPCRs that mediate slow and prolonged inhibitory action, which would be unaffected by application of the GABA-A antagonists.18 This hypothesis warrants further investigation. Pentylentrazole caused some changes to the pattern of electrical firing, but these changes are less clear compared to picrotoxin and bicuculline. We have also performed ion channel screening of the seizurogenic compounds against fifteen seizure-associated ion channels, as a complimentary method to identify seizure liability early. Pentylentrazole inhibited the GABA α1β2γ2 ion channel, so perhaps the lack of clear activity in our hiPSC cell model is due to our administration of the compound.
We used a single dose, whereas kindling models in vivo frequently use repeated doses to create epilepsy models.19 Enoxacin and amoxicillin did not inhibit the GABA α1β2γ2 ion channel at the clinically relevant doses used, suggesting that the epileptogenic mechanism of these compounds is not GABA-A related. No changes in electrical activity in hiPSC-neurons were observed, however seizurogenic responses have been reported in zebrafish and preclinical rodent models, suggesting a species related difference in enoxacin sensitivity.12,20,21
These observations highlight the importance of diligently interrogating cell models, considering the expression profile of the cells, and understanding the pharmacology and uses of test compounds. This allows scientists to gain a full understanding of their cell models, tweak their sensitivity, and understand what the model can detect, and more importantly, what the model is unable to detect, and why. Our approach to seizure liability screening is especially pertinent in the context of the recent FDA modernization act which allows applicants to use methods other than animal testing to establish drug safety and effectiveness. Our proposal for a human ion channel assay coupled with assessment of electrical activity in hiPSC neuronal cells fits these criteria,22,23 and has been highlighted by the FDA/CDER as a useful novel alternative method (NAM) that should be considered as a component of the overall safety assessment for seizure liability.. In addition, the development of our seizure liability assays has been presented at many conference.24 and has been recognised by several awards
Human neuronal cells: Possibilities in drug safety testing and beyond including the BioNow Rising Star award and honours relating to in vitro toxicology and drug-oriented toxicological research.25
The possibilities of this model don’t end at seizure detection. In addition to further refinement of this assay with respect to GABA-A/GABA-B, we also plan to further characterise the model for a liability that is the direct opposite of seizure – sedation. We have demonstrated that the hiPSC-neuronal cells show decreased activity with GABA agonists such as muscimol, and the benzodiazepine site agonist indiplon. Indiplon is a marketed sleeping aid whose decreased activity was immediately reversed with application of the benzodiazepine site antagonist, flumazenil, a compound that is used clinically to reverse benzodiazepine overdose.26 Outside of drug safety, we also plan to adapt our model to anti-epileptic drug discovery. This will be achieved by inducing a seizurogenic phenotype using a known seizurogenic compound, and then determining if these changes are reduced by application epilepsy treatments. Looking further afield to disease modelling, studies have been conducted using patient derived iPSC-neuronal cells to identify novel pathological mechanisms in several neurological conditions including Parksinson’s Disease, Alzheimer’s Disease and Fragile X Syndrome.27,28
Since the advent of hiPSC technology almost two decades ago enormous progress has been made in many areas of research.29 Within drug safety the development of hiPSC technology represents a paradigm shift as they allow us to assess the potential toxicities of new drugs on a readily available source of human cells – a situation that wasn’t possible before. With further characterisation and refinement of these models we will be able to streamline our drug safety testing, reduce reliance on animal models, and ultimately develop safer medicines.
Author Bio:
Dr Kimberley Rockley
Dr Kimberley is a Senior Scientist at ApconiX focused on the development of novel in vitro assays for early detection of seizure liability. She completed a PhD at Durham University which focused on investigating the mechanisms of cardiotoxicity of cancer therapies using clinically relevant in vitro human models of cardiac physiology paired with novel methodologies to evaluate structural and functional toxicities. Dr Rockley previously completed an MSc in Cancer Pharmacology and have experience in clinical research from an oncology clinical trial coordinator role.
References
- Blinova K, Stohlman J, Vicente J, Chan D, Johannesen L, Hortigon-Vinagre MP, et al. Comprehensive Translational Assessment of Human-Induced Pluripotent Stem Cell Derived Cardiomyocytes for Evaluating Drug-Induced Arrhythmias. Toxicol Sci. 2017 Jan;155(1):234–47.
- Gintant G, Fermini B, Stockbridge N, Strauss D. The Evolving Roles of Human iPSC-Derived Cardiomyocytes in Drug Safety and Discovery. Cell Stem Cell. 2017 Jul 6;21(1):14–7.
- Strauss DG, Gintant G, Li Z, Wu W, Blinova K, Vicente J, et al. Comprehensive In Vitro Proarrhythmia Assay (CiPA) Update from a Cardiac Safety Research Consortium / Health and Environmental Sciences Institute / FDA Meeting. Ther Innov Regul Sci. 2018;53(4):519–25.
- Colatsky T, Fermini B, Gintant G, Pierson JB, Sager P, Sekino Y, et al. The Comprehensive in Vitro Proarrhythmia Assay (CiPA) initiative — Update on progress. J Pharmacol Toxicol Methods. 2016 Sep 1;81:15–20.
- Cook D, Brown D, Alexander R, March R, Morgan P, Satterthwaite G, et al. Lessons learned from the fate of AstraZeneca’s drug pipeline: A five-dimensional framework. Nat Rev Drug Discov. 2014;13(6):419–31.
- Morgan P, Brown DG, Lennard S, Anderton MJ, Barrett JC, Eriksson U, et al. Impact of a five-dimensional framework on R&D productivity at AstraZeneca. Nat Rev Drug Discov. 2018 Mar;17(3):167–81.
- Authier S, Arezzo J, Delatte MS, Kallman MJ, Markgraf C, Paquette D, et al. Safety pharmacology investigations on the nervous system: An industry survey. J Pharmacol Toxicol Methods. 2016;81:37–46.
Human neuronal cells: Possibilities in drug safety testing and beyond - Walker AL, Imam SZ, Roberts RA. Minireview Drug discovery and development : Biomarkers of neurotoxicity and neurodegeneration. 2018;1037–45.
- Preuss TM. Taking the measure of diversity: comparative alternatives to the model-animal paradigm in cortical neuroscience. Brain Behav Evol. 2000 Jun;55(6):287–99.
- Scharfman HE. The Neurobiology of Epilepsy. Curr Neurol Neurosci Rep. 2007 Jul;7(4):348–54.
- Johnstone AFM, Gross GW, Weiss DG, Schroeder OHU, Gramowski A, Shafer TJ. Microelectrode arrays: a physiologically based neurotoxicity testing platform for the 21st century. Neurotoxicology. 2010 Aug;31(4):331–50.
- Easter A, Bell ME, Damewood JR, Redfern WS, Valentin JP, Winter MJ, et al. Approaches to seizure risk assessment in preclinical drug discovery. Drug Discov Today. 2009 Sep;14(17–18):876–84.
- Fan J, Thalody G, Kwagh J, Burnett E, Shi H, Lewen G, et al. Assessing seizure liability using multi-electrode arrays (MEA). Toxicol In Vitro. 2019 Mar 1;55:93–100.
- Liu J, Laksman Z, Backx PH. The electrophysiological development of cardiomyocytes. Adv Drug Deliv Rev. 2016 Jan 15;96:253–73.
- Rudy B, McBain CJ. Kv3 channels: voltage-gated K+ channels designed for high-frequency repetitive firing. Trends Neurosci. 2001 Sep;24(9):517–26.
- Gutman GA, Chandy KG, Adelman JP, Aiyar J, Bayliss DA, Clapham DE, et al. International Union of Pharmacology. XLI. Compendium of Voltage-Gated Ion Channels: Potassium Channels. Pharmacol Rev. 2003;55(4):583–6.
- Bradley JA, Luithardt HH, Metea MR, Strock CJ. In Vitro Screening for Seizure Liability Using Microelectrode Array Technology. Toxicol Sci. 2018;163(1):240–53.
- TERUNUMA M. Diversity of structure and function of GABAB receptors: a complexity of GABAB-mediated signaling. Proc Jpn Acad Ser B Phys Biol Sci. 2018 Dec 11;94(10):390–411.
- Shimada T, Yamagata K. Pentylenetetrazole-Induced Kindling Mouse Model. J Vis Exp. 2018;(136):56573.
- Tukker AM, van Kleef RGDM, Wijnolts FMJ, de Groot A, Westerink RHS. Towards animal-free neurotoxicity screening: Applicability of hiPSC-derived neuronal models for in vitro seizure liability assessment. Altex. 2020 Jan 1;37(1):121–35.
- Winter MJ, Redfern WS, Hayfield AJ, Owen SF, Valentin JP, Hutchinson TH. Validation of a larval zebrafish locomotor assay for assessing the seizure liability of early-stage development drugs. J Pharmacol Toxicol Methods. 2008;57(3):176–87.
- Roberts R, Authier S, Mellon RD, Morton M, Suzuki I, Tjalkens RB, et al. Can we panelize seizure? Toxicol Sci. 2021 Jan 1;179(1):3–13.
- Rockley KL, Roberts RA, Morton MJ. Innovative models for in vitro detection of seizure. Toxicol Res. 2019 Nov 1;8(6):784–8.
Human neuronal cells: Possibilities in drug safety testing and beyond - Avila AM, Bebenek I, Mendrick DL, Peretz J, Yao J, Brown PC. Gaps and challenges in nonclinical assessments of pharmaceuticals: An FDA/CDER perspective on considerations for development of new approach methodologies. Regul Toxicol Pharmacol RTP. 2023 Mar;139:105345.
- Rockley KL. LinkedIn Profile [Internet]. [cited 2023 Jun 15]. Available from: https://www.linkedin.com/in/kimberly-rockley-14884712a/
- Alexander SPH, Mathie A, Peters JA. Guide to Receptors and Channels (GRAC), 3rd edition. Br J Pharmacol. 2008 Mar;153 Suppl 2(Suppl 2):S1-209.
- Little D, Ketteler R, Gissen P, Devine MJ. Using stem cell-derived neurons in drug screening for neurological diseases. Neurobiol Aging. 2019 Jun;78:130–41.
- Rivetti di Val Cervo P, Besusso D, Conforti P, Cattaneo E. hiPSCs for predictive modelling of neurodegenerative diseases: dreaming the possible. Nat Rev Neurol. 2021 Jun;17(6):381–92.
- Shi Y, Inoue H, Wu JC, Yamanaka S. Induced pluripotent stem cell technology: a decade of progress. Nat Rev Drug Discov. 2017 Feb;16(2):115–30.
Related topics
Drug Discovery, Drug Discovery Processes, In Vitro, Neurons
Related organisations
ApconiX
Related people
Dr Kimberly Rockley (ApconiX)