Patient-derived organoids in disease modelling
Posted: 16 October 2023 | Taylor Mixides (Drug Target Review) | No comments yet
In an exclusive interview with Nikki Carter at Molecular Devices, we explore the the power of patient-derived organoids (PDOs) in drug discovery.
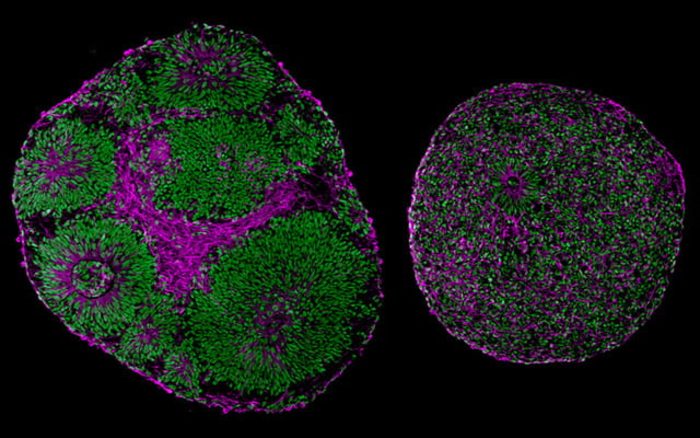
Microscopy images reveal significant differences in size and structure between brain organoids derived from a patient with Pitt-Hopkins Syndrome (right) and from a control (left) [Credit: UC San Diego Health Sciences].
These “patient in a dish” models, as explained by Molecular Devices, are three-dimensional (3D) cell structures cultivated from tissue samples that offer an opportunity to bridge the gap between traditional two-dimesion (2D) cell cultures and animal models. As we explore translatability, technological advancements and the integration of artificial intelligence (AI), we envision the potential role of PDOs throughout the entire medicine creation pipeline, from target identification to personalised clinical treatments, ultimately reducing costs and improving patient outcomes.
How can PDOs revolutionise drug discovery and deepen our understanding of disease?
Patient-derived organoids (PDOs) are proliferative 3D cell structures derived from tissue samples of both healthy and diseased tissue. They self-assemble and in doing so retain the genetics, structure and heterogeneity of cell types found in the tissue they derive from. Their close resemblance to the patient and disease state makes PDOs a valuable and scalable source of biological material to interrogate in disease research and drug discovery. These human translatable models are often termed ‘patient in a dish.’
What sets PDOs apart from traditional 2D cell and animal models used in drug discovery?
Improved translatability to the patient and the disease state. Two-dimensional cell models were the cornerstone of cellular assays in early drug discovery for years, yet the system lacks the genetic and epigenetic background of the patient and the cell-to-cell spatial architecture and cellular signalling between cells of different types within the tissue architecture. 2D cell models are often engineered to over-express the target of interest and so lack its temporal behaviour in its native or disease state. While PDOs do not precisely recapitulate all these factors, they are certainly more translatable than 2D models.
As for animal models, I am sure we all agree that reducing animal use in drug discovery is the ethical route forward. Just as importantly, there are areas of human physiology and disease where an animal model simply is not appropriate due to species differences. This is particularly pertinent in immune-based cell therapies like CAR-T, where animals do not mimic the human immune response. In orphan and rare diseases, it is hard to get an animal model to represent a disease state that is poorly understood. In many cases, a PDO is deployed to better effect than an animal because it makes a closer match between model and patient. This was recognised in the FDA Modernization Act 2.0, which recently removed the mandate for all drugs to be first tested on animals.
What technological advancements related to PDOs are refining disease modelling and drug screening?
Technology supporting the use of PDOs in the medicine pipeline is evolving fast and overcoming some of the barriers to their adoption. PDOs are used in functional genomics screening to model disease and drive target identification. In this method, PDOs representing the patient and disease state are CRISPR-modified to knock out genes systematically. This causes some organoids to not survive while others display phenotypic changes – each response is linked to the gene and used to find new mechanisms to target in the patient population.
How might PDOs realise their full potential?
I foresee the use of PDOs throughout the medicine creation pipeline, from target identification and discovery, to drug repurposing, right through to the clinic. By starting as early as possible in the drug discovery process with a cellular model that best represents the patient’s disease state, researchers will avoid the lack of clinical efficiency that is the key driver for attrition. And by using the patient’s organoid for educated prescribing decisions in complex diseases like cancer, clinicians will reduce treatment costs and ensure the most effective treatment for that patient. The full potential of PDOs would be to shorten the time and costs associated with medicine creation, and then use PDO therapies to treat patients effectively.
About the author
Nikki Carter
Commercial Organoid Innovation Director, Molecular Devices.
Nikki drives adoption of 3D cellular models of human disease by applying her expertise in cell biology to drug discovery and high-throughput screening. Nikki’s life sciences experience spans leadership, commercial and research roles.
Related topics
Drug Development, Drug Discovery Processes, Drug Targets, Organoids
Related organisations
Molecular Devices
Related people
Nikki Carter (Molecular Devices)