Inhibition of RNA-containing viruses by artificial RNases
Posted: 16 June 2016 | Dmitry Klinov (Federal Medical and Biological Agency of the Russian Federation), Elena Isaeva (D. I. Ivanovsky Institute of Virology), Ludmila Koroleva (Siberian branch of the Russian Academy of Sciences), Olga Morozova (D. I. Ivanovsky Institute of Virology and Federal Medical and Biological Agency of the Russian Federation), Vladimir Silnikov (Siberian branch of the Russian Academy of Sciences) | No comments yet
The toxicity and antiviral properties of 24 low-molecular-weight artificial RNases (artRNases) including peptides, peptidomimetics, N-alkyl-substituted 1,4-diazabicyclo[2.2.2]octane (DABCO) and a silver (I) complex with cystine were analysed for viruses containing single-stranded and double-stranded genomic RNA (the tick-borne encephalitis virus; TBEV and the influenza virus, respectively) in vitro and in vivo by both conventional methods and biosensor-mediated label-free optical detection.
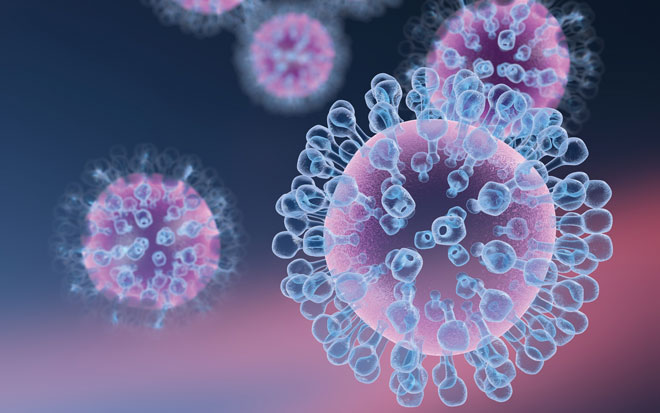
The biosensor was based on long-range surface waves on a one-dimensional photonic crystal surface in microfluid channels, which was able to detect binding of the viruses and their host cells with antivirals. Screening of toxicity of the artRNases in vitro showed that the most toxic were DABCO derivatives (cytotoxic concentrations СС50=0.0001-0.5 мМ), whereas peptides were less toxic (СС50=0.02-10 мМ).
Toxicity of the artRNases in mice appeared to depend on the route of administration, with the highest permissible doses after multiple peroral administrations. Complete cleavage of total RNA isolated from the infected cells – and only partial degradation of the viral RNA in virions or inside infected cells in the presence of the artRNases without qualitative and quantitative changes of the viral protein antigens – were found. Antiviral properties against the TBEV were revealed only for DABCO derivatives with remaining infectivity. Comparison of conventional methods to estimate toxicity and antiviral properties versus the label-free real time optical detection allowed us to suggest a high-throughput screening of new drugs.
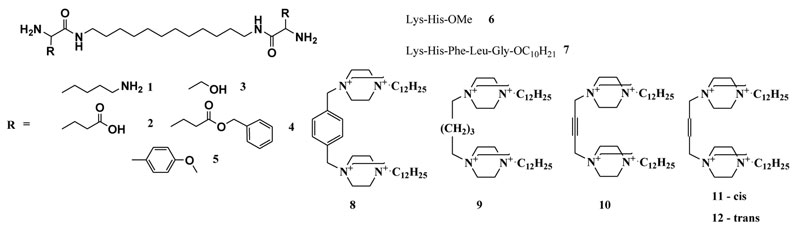
Figure 1: Artificial ribonucleases of different types: peptidomimetics (1-5) peptides (6-7), and polycationic DABCO derivatives (8-12)
Introduction
Since spontaneous mutagenesis and quasispecies rearrangements of RNA-containing viruses, as well as an absence of both viral and cellular RNA reparation systems, cause a virus to become resistant to originally effective antiviral drugs, combined therapy with inhibitors of viral enzymes and immunomodulators is recommended1,2,3. Specific immunoglobulins do not ensure complete virus elimination but rather may cause antibody-dependent enhancement of flavivirus infection by means of increased penetration of complexes of virions with specific antibodies into cells with receptors for Fc-fragments of immunoglobulins. Currently, the high mutation rate of RNA-containing viruses exceeds the limited repertoire of available antivirals. Therefore, a search for novel medications for combined therapy of viral infections or for inactivated vaccines remains a public health concern1,2,3.
The universal molecular target of the RNA-containing viruses is their genomic RNA. However, the natural protein RNases ubiquitous in body fluids cannot penetrate into either host cells or enveloped viruses despite the wide range of their molecular weights from 12 to 150 kDa4 and are therefore excluded from consideration as potential drugs. The protective role of RNase in milk against retroviral infection has been envisaged4. RNA-containing orthomyxoviruses seem to be sensitive to the elevated levels of RNases in blood5. Therefore, research into artRNases capable of penetrating virions and cells remains relevant6-21.
Unspecific artRNases include both metal-free compounds and complexes of transition metals (Cu2+ 6, Zn2+ 7, Ln3+ 8, Eu3+ 9) with organic ligands, biogenic amines10, some peptides11-12 and other low molecular weight organic compounds13-15 capable of cleaving phosphodiester bonds of certain dinucleotides (CpA and UpA). Site-specific artRNases are based on antisense oligonucleotides16, 20 and ribozymes17-19. The artRNases can inactivate non-enveloped RNA-containing viruses21. Our aim was to compare the toxicity and antiviral properties of the artRNases against the enveloped RNA-containing viruses using conventional approaches and microfluidic optical detection.
Materials and methods
Porcine embryo kidney (PS) cells; green monkey kidney Vero cells; Madin-Darby Canine Kidney (MDCK) epithelial cells and mouse subcutaneous connective tissue L929 were obtained from the Russian Tissue Culture Collection (Federal Research Center of Epidemiology and Microbiology of N.F. Gamaleya of the Russian Ministry of Health, Moscow, Russia) and grown in Eagle minimal essential medium (EMEM) supplemented with 10% foetal bovine serum in the presence of 100U/ml penicillin and 100U/ml streptomycin.
Influenza A virus of subtypes H1N1 (strain A/swine Iowa 15/30) and H3N2 (strain A/Aichi/1/68), the TBEV of the Far Eastern subtype (strain Sofjin), the Siberian subtype (strain Aina/1448) and the European subtype (strain Absettarov) were obtained from the Russian State Collection of Viruses (Federal Research Center of Epidemiology and Microbiology of N.F. Gamaleya of the Russian Ministry of Health, Moscow, Russia).
The artificial RNases had been synthesised as previously described11-13. Their structures were confirmed by means of infrared, ultraviolet light, nuclear magnetic resonance spectroscopy and data of element analysis.
Total RNA were isolated from control and infected cells22. Isolated RNA before and after incubation with the artRNases were analysed by electrophoresis in SDS-agarose gels with subsequent ethidium bromide staining.
Cleavage of nucleic acids
ArtRNases were dissolved in RNase-free sterile de-ionised water immediately before use. The isolated total RNA (5-10µg in 5µl) was incubated with 5µl of the artRNase solutions at 37°C for one hour. Following this, reaction products were assayed in reverse transcription with random N6 primers with subsequent real-time polymerase chain reaction with SyberGreen I and primers specific to the influenza A virus: InAA-F 5′- TCTGTCTGGCTCTCGGCC -3’ and InAA-R 5′- GATTGTTGCATATTTTCCCCG-3’, as well as primers and fluorescent hydrolysis probes specific to the TBEV: TBEVF 5’-YTTCAGACAGGAACCAACAC-3’; TBEVR 5’-CAGTTYCTCARGTCAGTGAC-3’ and TBEV-probe 5’-FAM-CATGGCGGTCCACACTGACCAAA-BHQ1-3’.
The influenza virus antigens were revealed in an enzyme-linked immunosorbent assay (ELISA) with immobilised polyclonal antibodies, secondary antibodies against mouse IgG with horseradish peroxidase with subsequent orthophenylendiamine staining23. The haemagglutination titers were determined using 0.75% human erythrocytes (blood group O)24.
Real time optical detection
Photonic crystal (PC)25-27 (http://pcbiosensors.com) was sonicated and treated in plasma cleaner. Functionalisation of the PC included 0.1mg/ml polyallylamine (pAA) 65 and 0.1% glutaraldehyde. Following the baseline stabilisation, solutions of proteins or artRNases as well as cellular or viral suspensions were added in a microfluid channel of the biosensor.
Subsequent 10-times dilutions of the artRNases solutions were added in quadruplicate in 48-well plates with the monolayer cells. To estimate toxicity two methods were used: MTT and label-free biosensor detection.
The fresh solutions of the artRNases were administered into adult mice (of ICR strain) in three ways:
- peroral administrations of 25ml 160mM solutions five times each day with intervals of one day between administrations;
- intranasal administration of 50ml per mouse of 20mM, 2mM, 0.2mM and 0.02mM solutions;
- subcutaneous injections of 100ml per mouse of 20mM, 2mM, 0.2mM and 0.02mM solutions.
Toxicity of the artRNases in mice was estimated on the basis of lethality as well as external signs such as body mass reduction, inflammation, physical activity, behaviour, absence of tremor, paresis, spasms and convulsions compared with control animals during the 20 days following administrations of the artRNases. Maximal nontoxic doses corresponded to half doses of the artRNases which did not cause noticeable consequences in the mice.
MDCK cells were infected with the influenza virus according to reference 28. The virus was detected using haemagglutination test24, ELISA 23 and reverse transcriptase-PCR.
Infection of ICR mice
Mice ICR (males, 10-12g) were intranasally infected under light ether anaesthesia with 50ml of the influenza A/Aichi 1/68 virus adapted to the mouse lungs (10 lethal dose LD50)29. The infected mice were observed for 20 days postinfection.
The freshly prepared solutions of the artRNases were added to wells with confluent monolayers of MDCK cells two hours before or after their infection with the influenza virus or TBEV. Microscopic observations of cytopathic effect caused by the viral infections continued over 48 hours29.
The solutions of the artRNases were administered perorally in adult mice. Both single and multiple administrations according to prophylactic and therapeutic schemes were performed. The treated and infected mice were observed for 21 days post-infection. Continuous variables were compared using Student’s t-test30. A P value of <0.05 was assumed to be significant.
Results and discussion
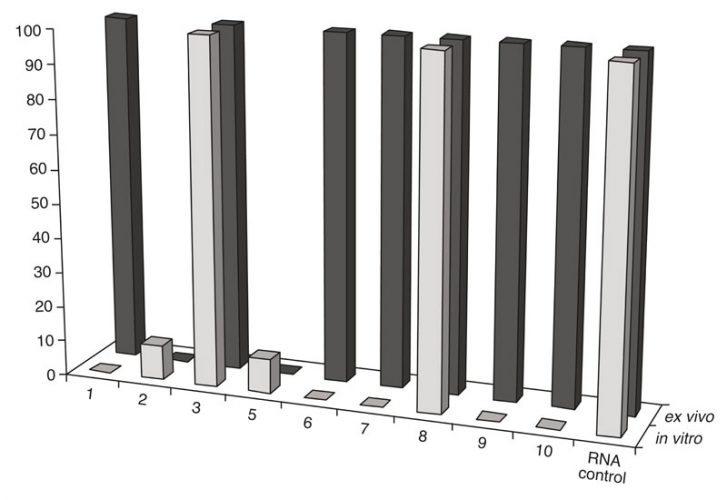
Figure 2: Comparison of RNA cleavage activity of the artRNases after their incubation in vitro with the isolated TBEV RNA and ex vivo (in purified extracellular virions)
The design, synthesis and estimation of nuclease activity of 24 artRNases have been previously described11-13; 31-33. They include peptidomimetics based on 1,12-diaminododecane and natural (lysine, serine and glutamine) or artificial amino acids1-5; peptides – methyl ester of dipeptide Lys-His6 and decyl ester of pentapeptide Lys-His-Phe-Leu-Gly7; 1,4-diazabicyclo[2.2.2]octane (DABCO) with different linkers8-12 as well as water-soluble silver (I) complex with cystine Li+[Ag+2Cys2–(OH–)2(NH3)2] (part of them is shown in Figure 1).
The most toxic compounds for four tissue cultures appeared to be DABCO derivatives with CC50 in a range 0.0001-0.5мМ (Table 1). CC50 values for peptides and peptidomimetics were 0.02-10мМ (Table 1). In vivo toxicity appeared to depend on the route of administration. One should note that multiple peroral administrations of the artRNases were not toxic for adult mice. Discrepancy between toxicity of the compounds in vitro and in vivo might be explained by their accumulation in organs of mice and different detoxication mechanisms.
RNA cleavage
Exhaustive cleavage of both cellular and viral-isolated RNA was revealed for one peptidomimetic 1 (A-336), both peptides6-7 and two DABCO derivates9-10 after their incubation with 1mM solutions of the artRNases for two hours at 370C (Figure 1). Full degradation of the influenza virus RNA only within extracellular virions required additional incubation for three hours. However, the TBEV RNA in virions was degraded only in the presence of the peptidomimetics 2 and 5 (Figure 2). Neither cellular DNA nor viral antigens were damaged after incubation with the artRNases, as shown by means of the biosensor-mediated optical detection (Figure 3), ELISA, hemagglutination test and real-time PCR.
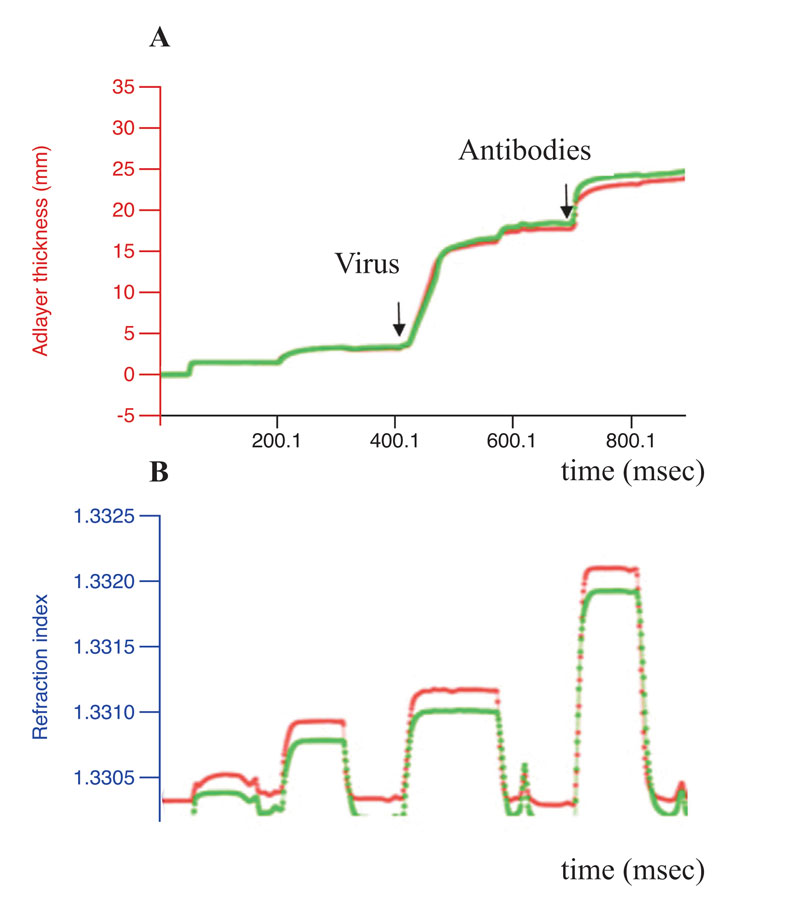
Figure 3: Conformational stability of the influenza virus antigens. Results of the label-free real-time optical detection of binding of the influenza A virus before (green curve)
The artRNases inhibited the influenza virus more efficiently than the TBEV, perhaps due either to differing permeability of the viral envelopes or distinct secondary structures of the genomic RNA and their encapsidation. TBEV inactivation was not complete. Difference between the virus titers before and after treatment with the artificial RNases were 2-3 lg TCID50.Therefore, the approach cannot be recommended either for vaccine preparation or for TBE treatment.
Taking into consideration the relatively low toxicity and evident anti-influenza virus properties with RNA as a new molecular target, one could conclude that the novel low-molecular-weight RNases without immunogenic and allergenic activities might be used for an inactivated vaccine preparation and for combined therapy of influenza. The presence of both viral RNA cleavage fragments and protein antigens in native conformations might induce a balanced protective immune response.
References
- Baier A. Flavivirus infections and potential targets for antiviral therapy. In: Ruzek D., ed. Flavivirus encephalitis. Intech, Croatia; 2011: 89-104
- De Clercq Antiviral agents: characteristic activity spectrum depending on the molecular target with which they interact. Adv. Virus Res. 1993; 42: 1-55
- De Clercq E. Highlights in antiviral drug research: antivirals at the horizon. Med. Res. Rev. 2013; 33(6): 1215-48
- Ramaswamy H, Swamy ChVB, Das MR. Purification and characterization of a high molecular weight ribonuclease from human milk. J Biol Chem 1993;268:4181-4187
- Smirnov IuA, Kolodkina VP, Kaverin NV. Action of hydrolytic enzymes on influenza virus A ribonucleoprotein. Vopr Virusol. 1981;4:477-481 (In Russian)
- Young MJ, Chin J. Dinuclearcopper(II) complex that hydrolyzes RNA. Am Chem Soc. 1995;117:10577-10578
- Boseggia E, Gatos M, Lucatello L, Mancin F, Moro S, Palumbo M, Sissi C, Tecilla P, Tonellato U, Zagotto G. Toward efficient Zn(II)-based artificial nucleases. Am Chem Soc. 2004;126:4543-4549
- Gunnlaugsson T, O’Brien JE, Mulready S. Glycine-alanine conjugated macrocyclic lanthanide ion complexes as artificial ribonucleases. Tetrahedron Lett. 2002;43:8493-8497
- Komiyama M, Sumaoka J. Progress towards synthetic enzymes for phosphoester hydrolysis. Curr Opin Chem Biol. 1998;2:751-757
- Fouace S, Gaudin C, Picard S, Corvaisier S, Renault J, Carboni B, Felden B. Polyamine derivatives as selective RNaseA mimics. Nucleic Acids Res. 2004;32:151-157
- Zhdan NS, Kuznetsova IL, Vlassov AV, Sil’nikov VN, Zenkova MA, Vlassov VV. Synthetic ribonucleases. 1. Synthesis and properties of conjugates, containing an RNA-binding fragment based on lysine residues and an RNA hydrolyzing fragment, bearing an imidazole residue. Bioorg Khim. 1999;25:723-732
- Koroleva LS, Donina AA, Tamkovich NV, Kovalev NA, Zenkova MA, Sil’nikov VN. Artificial ribonucleases 6. Ribonuclease activity of tetrapeptides based on amino acids involved in the catalytic site of RNase T1. Russ Chem Bull. 2005;54:2682-2691
- Konevetz DA, Beck IE, Beloglazova NG, Sulimenkov IV, Sil’nikov VN, Zenkova MA, Shishkin GV, Vlassov VV. Artificial ribonucleases: synthesis and RNA cleaving properties of cationic conjugates bearing imidazole residues Tetrahedron 1999;55:503-512
- Scheffer U, Strick A, Ludwig V, Peter S, Kalden E, Göbel MW. Metal-free catalysts for the hydrolysis of RNA derived from guanidines, 2-aminopyridines, and 2-aminobenzimidazoles. J Am Chem Soc. 2005;127:2211-2217
- Kovalev N, Burakova E, Silnikov V, Zenkova M, Vlassov V. Artificial ribonucleases: from combinatorial libraries to efficient catalysts of RNA cleavage. Bioorg Chem. 2006;34:274–286
- Niittymäki T, Lönnberg H. Artificial ribonucleases. Org Biomol Chem. 2006;4:15-25
- Vorobjeva M, Gusseva E, Repkova M, Kovalev N, Zenkova M, Venyaminova A, Vlassov V. Modified binary hammerhead ribozymes with high catalytic activity. Nucleosides Nucleotides Nucleic Acids 2005;24:1105-1109
- Vorobjeva M, Zenkova M, Venyaminova A, Vlassov V. Binary hammerhead ribozymes with improved catalytic activity. Oligonucleotides 2006;16:239-252
- Vorobjeva M, Zenkova M, Venyaminova A, Vlassov V. Binary hammerhead ribozymes with improved catalytic activity. Oligonucleotides 2006;16:239-252
- Gamble C, Trotard M, Le Seyec J, Abreu-Guerniou V, Gernigon N, Berrée F, Carboni B, Felden B, Gillet R. Antiviral effect of ribonuclease conjugated oligodeoxynucleotides targeting the IRES RNA of the hepatitis C virus. Bioorganic and Medicinal Chem Lett. 2009;19:3581–3585
- Fedorova AA, Azzami K, Ryabchikova EI, Spitsyna YE, Silnikov VN, Ritter W, Gross HJ, Tautz J, Vlassov VV, Beier H, Zenkova MA. Inactivation of a non-enveloped RNA virus by artificial ribonucleases: Honey bees and Acute bee paralysis virus as a new experimental model for in vivo antiviral activity assessment. Antiviral Research 2011;91:91267–91277
- Chomczynski P, Sacchi N. Single-step method of RNA isolation by acid guanidinium thiocyanate-phenol-chloroform extraction. Anal Biochem. 1987;162:156-159
- Isaeva EI, Rovnova ZI, Selivanov YaМ, Identification of the hemagglutinating antigens of the influenza virus by immunoenzyme analysis. Vopr. virusol. 1988;5:538-543
- Zdrodovskyi PF, Sokolova MI, Manual for laboratory diagnostics of viral and rickettsial diseases. Moscow: Medicine; 1965
- Konopsky VN, Alieva EV. Optical biosensors based on photonic crystal surface waves. Methods Mol Biol. 2009;503:49-64
- Konopsky VN, Alieva EV. A biosensor based on photonic crystal surface waves with an independent registration of the liquid refractive index. Biosens Bioelectron. 2010;25:1212-1216
- Konopsky VN, Karakouz T, Alieva EV, Vicario C, Sekatskii SK, Dietler G, Photonic crystal biosensor based on optical surface waves. Sensors (Basel) 2013;13:2566-2578
- Davies HW, Appleyard G, Cunnighan P, Perera MS. The use of continuous cell line for the isolation of influenza virus. Bull.WHO. 1978;56:1991-1993
- Reed L, Muench H. A simple method of estimating 50% endpoints. Amer. J. Hygiene 1938;27:493–497
- Lakyn GF. Biometry. Moscow: High School; 1974
- Gulevich AV, Koroleva LS, Morozova OV, Bakhvalova VN, Silnikov VN, Nenajdenko VG. Multicomponent synthesis of artificial nucleases and their RNase and DNase activity. – Beilstein Journal of Organic Chemistry 2011;7:1135-1140
- Goncharova EP, Koroleva LS, Silnikov VN, Ternovoy VA, Vlassov VV, Zenkova MA. Inactivation of tick-borne encephalitis virus by RNA-cleaving compounds. J Mol Genet Med. 2011;5:266-272
- Morozova OV, Isaeva EI, Silnikov VN, Barinov NA, Klinov DV. Antiviral properties and toxicity of Ag-cystine complex. Journal of Virology and Emergent Diseases 2016; 2(1) https://www.sciforschenonline.org/journals/virology/current-issue.php
Biographies
Olga V. Morozova graduated from the Novosibirsk State University in 1984. Her PhD thesis in 1991 was devoted to tick-borne encephalitis virus replication using affinity modification. She is currently the leading Researcher of Federal Centers. Her scientific interests include molecular typing of RNA-containing viruses and analysis of antiviral drugs. Olga V. Morozova can be contacted at: [email protected]; tel: +7(916)4212628.
Dmitry Klinov received a Master of Science degree in Molecular Biophysics from Moscow Institute of Physics and Technology in Moscow, in 1994, and a PhD in Molecular Biophysics from Moscow Institute of Physics and Technology in Moscow, in 1997. He held the post-doctoral position in the Laboratory of Electron Microscopy, Shemyakin-Ovchinnikov Institute of Bioorganic Chemistry, Russian Academy of Sciences, Moscow, from 1997 and since 2002 he has held the Senior Staff Scientist position. Dr. Klinov has published full-length papers in top scientific journals such as Science, Nucleic Acid Research, Applied Physics Letters and Chembiochem on the scanning probe microscopy of biopolymers.
Elena I. Isaeva works at D.I. Ivanovsky Institute of Virology of the Federal Research Center of Epidemiology and Microbiology of N.F. Gamaleya of the Russian Ministry of Health in Russia.
Ludmila S. Koroleva and Vladimir N. Silnikov are based at the Institute of Chemical Biology and Fundamental Medicine of the Siberian Branch of the Russian Academy of Sciences in Russia.
Related topics
Label-Free, RNAs
Related organisations
D. I. Ivanovsky Institute of Virology, Federal Medical and Biological Agency of the Russian Federation, Siberian branch of the Russian Academy of Sciences
Related people
Dmitry Klinov, Elena Isaeva, Ludmila Koroleva, Olga Morozova, Vladimir Silnikov