Switching on genes regulating oxysterol synthesis – a therapeutic option?
Posted: 20 September 2016 | Dr Steve Meaney (Assistant Head of the School of Biological Sciences: Dublin Institute of Technology) | No comments yet
Cholesterol is a ubiquitous molecule that, while essential for health, is probably best known as a risk factor for cardiovascular disease. It is also the obligate precursor for a wide range of metabolites, from steroid hormones to bile acids. Although studied for more than 100 years, recently there has been a resurgence of interest in the study of oxygenated metabolites of cholesterol, known commonly as oxysterols…
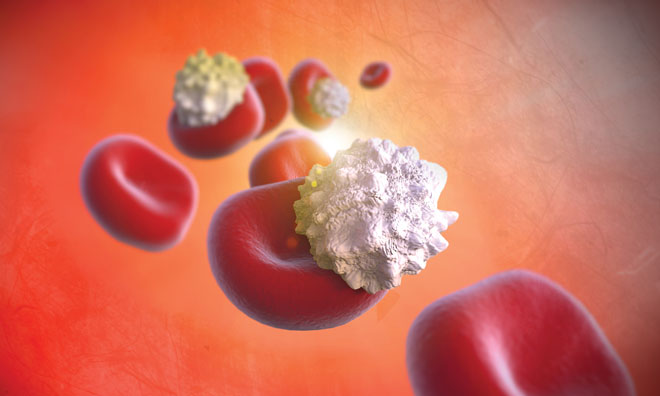
Cholesterol is a ubiquitous molecule that, while essential for health, is probably best known as a risk factor for cardiovascular disease. It is also the obligate precursor for a wide range of metabolites, from steroid hormones to bile acids. Although studied for more than 100 years, recently there has been a resurgence of interest in the study of oxygenated metabolites of cholesterol, known commonly as oxysterols. This is related to their activity as nuclear receptor ligands, metabolic regulators, immune modulators and regulators of cell growth. As additional details of the inter- and intracellular itineraries and physiological activities of individual oxysterols emerge, new opportunities to exploit enzyme pathways that govern synthesis of endogenous bioactive oxysterols will materialise in parallel. This article provides a brief overview of oxysterol biology and identifies potential avenues to translate this into new therapies.
Classically oxysterols are described as cholesterol metabolites with one or more additional oxygen functions such as hydroxyl, carbonyl, epoxy, hydroperoxy or carboxylate groups1. They may be formed via various enzymes encoded by genes regulating oxysterol synthesis (GROS), or by spontaneous, low-temperature auto-oxidation and modification, which may take place at virtually any site on the cholesterol skeleton. Recent research has identified that some sterol intermediates of cholesterol synthesis can also be considered as oxysterols.
From a biochemical viewpoint oxysterols are authentic intermediates in the bile acid biosynthesis pathway, the quantitatively dominating pathway for removal of cholesterol from the body, and have been studied extensively in this context1,2. Indeed, the introduction of an initial hydroxyl group is the initial step in a variety of different cholesterol elimination pathways active throughout the body (see Figure 1). As expected the content of oxysterols varies across the cells and tissues of the body, typically in parallel with the expression of the generative enzymes in that tissue. While not an absolute rule, typically the amount of a mono-oxygenated oxysterol such as 7α-, 24(S)- and 27-hydroxycholesterol present in a given tissue is significantly higher than their respective dihydroxylated metabolites. Moreover, work on immunomodulatory sterols indicates that, due to local differences in GROS expression, biologically relevant oxysterol gradients exist between different cell types in the same tissue3.
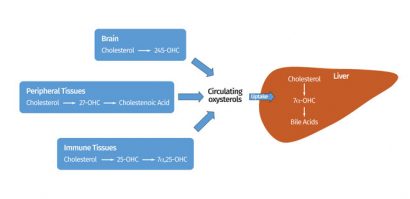
Figure 1: Oxysterols may be produced by many cells and tissues and terminal elimination is believed to be via metabolism in the liver, mainly to bile acids
Determinants of the availability of free oxysterol
As oxysterols are intermediates in cholesterol catabolism, the overall concentration of a given oxysterol in a tissue or cell is dependent on a number of competing processes – formation, modification (for example, by GROS or other enzymes), storage (e.g., as oxysteryl esters) or elimination from the generative cell. As an example, the oxysterol 24S-hydroxycholesterol (24S-OHC), a known and efficacious nuclear receptor ligand4 , is formed from brain cholesterol by the action of the neuron-specific cytochrome P450 enzyme cholesterol 24-hydroxylase (CYP46A1)5 . 24S-OHC rapidly exits the generative cells and traverses the blood-brain barrier6 . Once in the general circulation most of it becomes esterified to fatty acids by lecithin-cholesterol acyltransferase and incorporated into circulating lipoproteins.
Following hepatic uptake 24S-OHC is then either 7α-hydroxylated by 24S-hydroxycholesterol 7α-hydroxylase (CYP39A1) and fed into bile acid synthesis, or sulphated and glucuronidated and eliminated in the faeces7,8. Given that it is only the free sterol that is biologically active as a nuclear receptor ligand, it is evident that there are a variety of processes that reduce the availability of the free sterol, notwithstanding competition between the different oxysterol binding proteins within the cell. However, it is evident from preclinical in vivo studies that enhancing the production of endogenous metabolites such as 24S-OHC can drive changes in gene expression, physiology and disease development – at least in some studies9-11.
Oxysterols as ligands for nuclear receptors
It has been almost twenty years since oxysterols were identified as ligands for the liver-X-receptor (LXR) nuclear receptors4 . LXRα and LXRβ are important metabolic effectors that regulate a variety of important metabolic and inflammatory processes, including bile acid synthesis, cholesterol elimination, innate immunity and inflammation (reviewed elsewhere12,13). The most efficacious activators of the LXRs are believed to be side-chain oxidised sterols, such as 24S-OHC and 24S,25- epoxycholesterol (24S,25-EC). However, many other sterols, including 25-hydroxycholesterol (25-OHC) and desmosterol are able to bind to and activate LXRs under certain conditions14. Activation of LXRs typically leads to an increased capacity to eliminate cholesterol from the periphery via enhanced formation of HDL or from the liver via enhanced bile acid formation and transintestinal cholesterol excretion15. Several pharmaceutical companies are currently seeking to develop small molecule regulators of the LXRs and are at various stages in the process (most recently reviewed by Loren, J., et al.16). However, a consistent challenge has been uncoupling the beneficial effects (e.g., cholesterol elimination) from unwanted side effects (e.g., hepatic lipid accumulation driven by LXRα activation). These effects do not seem as prominent when activation is driven by endogenous ligands, although this remains to be systematically tested.
It is clear that oxysterols have many and varied roles, and alteration in the concentration of free sterol can have profound effects on cell physiology
The retinoic acid receptor-related orphan receptors (RORs) have been identified as an additional class of nuclear receptors with oxysterol ligands. 24S-OHC and 7-oxygenated oxysterols, in particular 7α-hydroxycholesterol (7α-OHC), appear to be high-affinity (low nanomolar Ka) inverse agonists for RORα and RORγ17,18. A very recent biochemical screen reported that a wide range of intermediates of cholesterol synthesis were able to interact with RORγ and activate it19. The importance of one late-stage intermediate, 4α-carboxy-4β-methyl zymosterol (called 4ACD8 by the authors and, as it carries a carboxylate group at the 4α-position, an oxysterol by the definition given above) was highlighted as a ligand for the thymus-specific RORγt and it appears to play a role in lymphocyte development – RORγt is required for the production of TH17 cells. Intriguingly, in patients deficient in SC4MOL (the enzyme responsible for the production of 4ACD8) immune dysregulation and psoriasis is present, in addition to the expected disturbances in cholesterol balance20. In addition, a dihydroxylated oxysterol 7β,27-dihydroxycholesterol (7β,27-diOHC) has also been identified as an agonist for RORγt21. Taken together these studies indicate a complex interplay of competing sterols for modulation of nuclear receptor activity.
Oxysterols as immunosterols
25-Hydroxycholesterol is one of the most studied oxysterols, largely due to its ready availability over the past few decades. In recent years it has emerged as a very potent regulator of the immune system and appears to have the ability to inhibit IL1B transcription (via well recognised activity on the processing of the sterol response element-binding protein (SREBP)) and activation (via effects on the inflammasome)22. 25-OHC thus acts as an immunosuppressant, presumably to ‘put the brakes’ on inflammatory responses at the local level. There is also good evidence that tolllike receptor activation leads to substantial increases in the production of 25OHC, rendering it temporarily the dominating oxysterol in the circulation – at least in the case of mice (where a 20-fold increase has been reported compared to a two-fold increase in the only available human data)23-25.
Oxysterols as ligands for GPCRs
The first identification of an oxysterol ligand for a G-protein coupled receptor (GPRC) was in 2011 where classical methods of biochemical fractionation were used to isolate activators of the orphan GPCR Epstein-Barr virus-induced gene 2 (EBI2)26,27. In contrast to the typically monooxygenated nuclear receptor ligands, the most potent EBI2 ligand was identified as the dihydroxylate 7α,25-dihydroxycholesterol (7α,25-diOHC) with 7α,27-dihydroxycholesterol only one-tenth as potent. Notably, neither 25-OHC nor 27-OHC alone had significant activity on EBI2. Via activation of EBI2 7α, 25-diOHC acts as a chemoattractant and an inability to generate this ligand results in an inability to mount appropriate plasma cell responses to immune challenges. Curiously, 7α- hydroxylation of 25-OHC is part of the normal catabolic pathway in the liver, whereby 25-OHC is converted into bile acids and 7α-hydroxylation is usually associated with inactivation of a sterol. In this case, however, inactivation is mediated by a dehydrogenation reaction where the 3β-hydroxy group is converted to a carbonyl group3 .
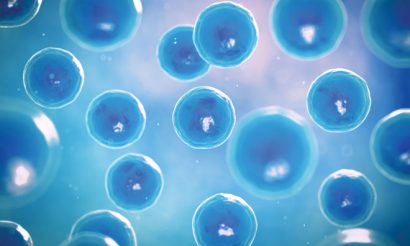
Altering concentrations of free sterols can have profound effects on cell physiology
Regulation of oxysterol formation as a therapeutic strategy
It is clear that oxysterols have many and varied roles, and alteration in the concentration of free sterol can have profound effects on cell physiology. The potential mechanisms for modulating the concentration are also evident from the biological activities described above and may be reduced to alteration of synthesis and alteration of metabolism. Using selected examples this section explores the concepts in which enzymes may be exploited to modulate free sterol concentration and oxysterol-dependent effects and signalling. For oxysterols that are derived from cholesterol, cytochrome P450 enzymes represent the major class of GROS while various other enzymes are involved in pathways leading to the production of oxysterols as part of cholesterol biosynthesis. GROS are relatively insensitive to classical regulators of P450 drug metabolism (e.g., carbamazepine), with the exception of CYP3A4 which can catalyse the formation of 4β-hydroxycholesterol from cholesterol (and according to one investigator, controversially, may also influence 25OHC)28-30.
As outlined above, CYP46A1 is responsible for the formation of 24S-OHC and enhancement of its expression using gene therapy and transgenic approaches has been shown to increase the amount of the 24S-OHC in vivo9,10. Compared with other cytochrome P450 GROS, CYP46A1 does not appear to be significantly regulated at the transcriptional level31. However, it has been clearly demonstrated that this gene is under epigenetic regulation and treatment with histone deacetylase inhibitors (HDACi) and/or DNA methyltransferase inhibitors results in a dramatic increase in transcriptional activity of this gene and, in some systems, an increase in 24S-OHC31. Various synthetic and natural HDACi are already available, although their efficacy in increasing the cellular content of 24S-OHC remains to be determined. A further complication is the potential effects on downstream metabolism, such as via the induction of CYP39A132. Thus, while this is relatively straightforward and, in the case of natural HDACi such as L-sulforaphane, attractive from a safety point of view, the off target effects may limit the effectiveness of this approach to increase oxysterol mediated regulation in vivo.
25-Hydroxycholesterol is one of the most studied oxysterols, largely due to its ready availability over the past few decades
An alternative approach that has yet to be explored is to inhibit or alter the activity of CYP39A1, which appears to be responsible for the majority of 24S-OHC metabolism and to which few biological activities have been ascribed. Blocking the metabolism of 24S-OHC should increase its concentration which would be expected to drive biological effects mediated by LXR and/or ROR. In the case that blocking CYP46A1 would be desirable, a direct inhibitor could be used (cf below), or enhancing the activity or expression of CYP39A1 (tissue specific epigenetic regulation of this gene in mice has been described). With regard to the ROR, as both 7α-OHC and 24S-OHC are active as inverse agonists, the 7α-24S-diOHC may be a more potent ligand, in a situation analogous to that of 7α,25-OHC and EBI2.
Modulation of GROS activity may also be used to alter the amount of available oxysterol and several inhibitory molecules have been described33-35. Importantly, several of these are existing drugs that would be amenable to repurposing in order to manipulate the GROS and so oxysterol levels. Both inhibitors and allosteric activators have been described. CYP46A1 is perhaps the best studied of these, due to its importance in significant neurodegenerative diseases such as Alzheimer’s disease, Huntington’s disease and others. Antifungals such as ketoconazole, iatraconazole and voriconazole – which were developed to target the CYP51A1 gene involved in cholesterol synthesis in mammals and ergosterol synthesis in fungi – are known inhibitors of GROS, although they are relatively non-specific.
Voriconazole and iatraconazole have been shown to modulate the plasma and brain levels of 24S-OHC in mice and plasma levels in man, respectively35,36. In vitro studies have defined voriconazole as an efficient inhibitor of CYP46A1 with a Ki of approximately 11nM35 and others reported CYP46A1 inhibitors include the drugs dexmedetomidine and fluvoxamine34. In addition to these, several possible activators of CYP46A1 activity have been described, including the antiretroviral efavirenz and the commonly used analgaesic acetaminophen37. Detailed studies of efavirenz have been carried out and it has been shown to potently increase activity in intact microsomes (by up to 11-fold), as well as influence the concentration of 24S-OHC in the brains of mice34. The potential engagement of LXR and related pathways were not explored in these animal models. The activity of acetaminophen is intriguing because it suggests that it would be possible to engineer relatively simple activators of CYP46A1 and perhaps other GROS that would be well tolerated and therapeutically useful.
Concluding remarks
The ever expanding role of oxysterols in human biology has placed a renewed focus on the potential to modulate their levels to enhance or suppress their endogenous effects. Small-molecule regulators of GROS have been described and these have the potential to increase endogenous oxysterols in a controllable way to achieve a defined endpoint. The capacity of oxysterols to act as both transport forms of cholesterol as well as regulators of metabolic and immunological processes underscores their role as ‘amplifiers’ essential for biology and with an ability to prevent diseases, such as neurodegenerative or cardiovascular disease.
Dr Steve Meaney is Assistant Head of the School of Biological Sciences, Dublin Institute of Technology, Ireland. He received his PhD in medical biochemistry from the Karolinska Institute in Stockholm and holds an MSc in Applied E-learning. His scientific interests include sterol biochemistry, both in terms of basic biology and in applications for controlling the production of noncholesterol sterols and modulating their levels therapeutically.