HTS assays for the identification of small-molecule inhibitors of deubiquitinating enzymes
Posted: 4 December 2016 | Elisabeth Weber (HelmholtzZentrum Munchen), Kamyar Hadian (HelmholtzZentrum Munchen), Kenji Schorpp (HelmholtzZentrum Munchen) | No comments yet
The covalent modification of proteins by the attachment of ubiquitin (ubiquitination) is best known for its function to label proteins for proteasomal degradation. However, ubiquitination also plays non-proteasomal roles during signal transduction, DNA repair and protein sorting…
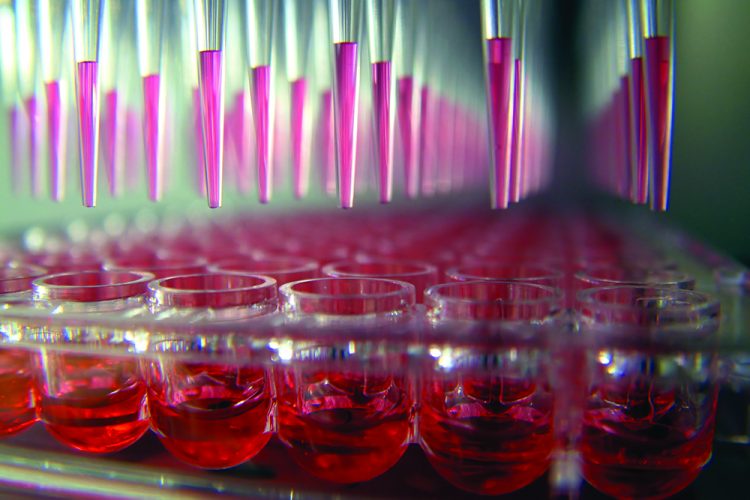
Many studies have shown that deregulation of the ubiquitin system is connected to the development of numerous diseases, emphasising how important proper control of this system is. In particular, deubiquitinating enzymes (DUBs), a large group of proteases, can regulate the stability of proteins, coordinate the cellular localisation and activate and inactivate proteins. This enzyme class has the ability to cleave ubiquitin chains and thereby reverse ubiquitin-dependent processes. Deregulation of certain DUBs has been implicated in various cancers, thereby making them an interesting drug target. In this article we will give an overview of different high-throughput screening (HTS)-applicable assay types that can be applied to drug discovery campaigns in order to identify small-molecule inhibitors of this enzyme class.
The role of DUBs within cellular processes
Ubiquitin (Ub) is a small regulatory protein modifier that can be attached to proteins, targeting them for destruction by the 26S proteasome1 or regulating a manifold of cellular functions2. Alterations of the ubiquitin system have been directly implicated in the development of many diseases. The 2004 Nobel Prize for Chemistry was awarded to Avram Hershko, Aaron Ciechanover and Irwin Rose for their discovery of ubiquitin-mediated protein degradation. More recently, in recognition of the central importance of protein and organelle degradation, the 2016 Nobel Prize in Physiology or Medicine was awarded to Yoshinori Ohsumi for his discoveries of the mechanisms for autophagy. Amongst others he identified that extension of the phagophore to form the mature autophagosome involves two Ub-like protein conjugation cascades3-4, again highlighting the central role of tight regulation of Ub-like systems.
Like many other protein modifications, ubiquitination is a reversible process. This deconjugation step is catalysed by the DUBs. DUBs can hydrolase isopeptide bonds between the C-terminus of Ub and a lysine side chain. This can lead to the removal of Ub from modified proteins, or the cleavage of polyUb-chains, in order to regulate Ub-dependent processes5. In summary, DUBs have three major functions: (i) cleaving freshly transcribed linear ubiquitin-fusion-chains into mono-Ub; (ii) removing Ub from post-translational modified proteins and thereby rescuing proteins from degradation; and (iii) altering the biological function of proteins by trimming Ub-chains and thereby controlling signalling processes. Thus, it is not surprising that DUBs play a critical role in diverse cellular processes.
Deregulation of DUBs can lead to imbalances of protein levels and changes in signalling pathways and hence is well defined as a cause of numerous human diseases6. Several DUBs have well-established links to cancer, mostly when their substrates are oncoproteins. Moreover, DUBs control the stability of multiple proteins involved in cellular proliferation and cell survival. For instance, USP7 is known to directly influence the stability of the tumour suppressor p53.7-8 Therefore, USP7 appears to be a promising, strategic target for cancer therapy and has been investigated in different small molecule HTS campaigns9-10. Familial cylindromatosis, an autosomal dominant predisposition to multiple neoplasms of the skin appendages, is another example of DUBs being directly involved in disease development. It has been shown that cylindromatosis has its genetic cause by mutations in the cyld tumour suppressor gene11 that ablates the DUB activity of CYLD12-14. Taken together, the well-characterised protease activity and the involvement of DUBs in several human diseases have led to an increasing interest from researchers to pharmacologically target this enzyme class15–16.
There are approximately 100 DUBs encoded in the human genome that have maintained remarkable specificity towards their protein substrates and ubiquitin chain types. Concerning their mechanism of action, DUBs are divided into five subclasses: ubiquitin specific proteases (USP), ubiquitin C-terminal hydrolyses (UCH), Josephine domain proteases, ovarian tumour proteases (OTU) and JAB1/MPN/MOV34 (JAMM) metallo-enzyme proteases17. The standard and classic setting to study DUB activity, linkage specificity and potency of DUB enzyme inhibitors is with in vitro DUB assays, which use native and unlabelled recombinant Ub-chains and recombinantly purified proteins. To this end, DUBs are incubated with di- or tetra-Ub substrates of different linkage-types and the reactions are usually analysed via SDS-PAGE and Western Blot18. However, this assay uses low-throughput methodology and requires relatively large amounts of enzymes and substrates.
As pharmacological inhibition of DUBs increasingly becomes the focus of researchers, there is a growing need of HTS-applicable methods for small molecule identification. In recent years great efforts have been undertaken to develop those assays. The following section gives a brief overview of the current state-of-the-art assays, their advantages and drawbacks. These assays are summarised in Table 1 and some of them are illustrated in Figure 1.
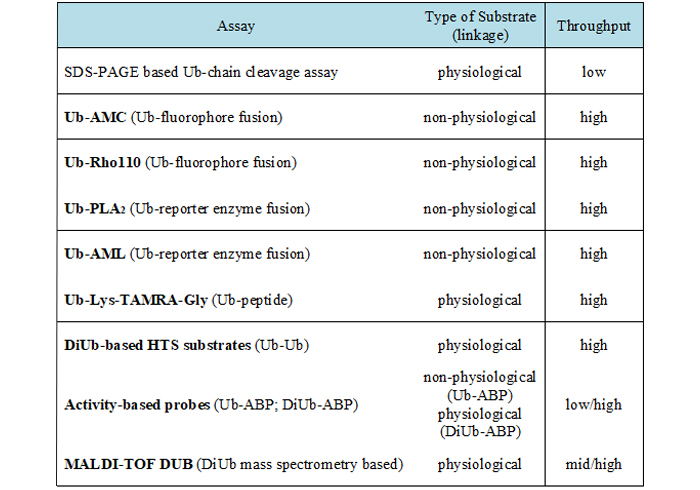
Table 1: Summary of the various DUB assay types
HTS-compatible DUB assays
Non-physiological Ub-Fluorophore fusions
Ub-AMC
A frequently used fluorogenic substrate is ubiquitin coupled via its C-terminus onto the amino group of the fluorescent tag 7-amino-4-methylcoumarin (Ub-AMC)19. DUBs can hydrolyse Ub-AMC and the liberation of highly fluorescent AMC allows steady-state kinetic parameters and mechanistic aspects of catalysis in a plate reader format to be analysed (Figure 1). As apparent for fluorescent screening technologies, the AMC-assay is prone to select fluorescent artifacts. Ub-AMC is an UV-active fluorophore and excitation in the UV-range is known to excite a number of screening compounds.
Ub-Rho110
While Ub-AMC can select for false positive fluorescent compounds, the use of the rhodamine (Rho) fluorophore with a red-shifted readout can reduce this risk and can thereby be a viable option20. The principle is equivalent to Ub-AMC, where the rhodamine is coupled to Ub and the cleavage leads to generation of fluorescence. Here, the disubstituted rhodamine moiety in Ub-Rho110 is essentially non-fluorescent and cleavage results in a mono-substituted rhodamine (Rho110), which exhibits intense fluorescence when excited at 485nm.
Non-physiological Ub-reporter enzymes
Ub-PLA2
Instead of fluorophores it is also possible to utilise a reporter enzyme (PLA2) conjugated to the C-terminus of Ub. PLA2 is inactive in its conjugated form, as it requires a free amino terminus. However, it becomes catalytically active when the Ub-PLA2 bond is hydrolysed21. Free PLA2 is subsequently able to turn over its substrate in a Ca2+-dependent reaction, thereby releasing a fluorescent product.
Ub-AML
The Ub-AML reagent consists of a Ub molecule linked to amino-luciferin (AML) at its C-terminal end. DUBs can cleave the linear fusion to release free AML. Importantly, AML is not luminescent by itself and light is only emitted when non-conjugated AML gets into complex with luciferase (Figure 1). The luciferase enzyme acts on free AML in the presence of ATP and Mg2+, leading to oxidation and thereby the release of photons that are detected in a plate reader22.
Physiological isopeptide-based assay reagents
A drawback of non-physiological HTS Ub-substrates is that they do not contain isopeptide bonds. These assays can be used in large screening campaigns and may deliver potential inhibitors of DUBs; however, these hits might not inhibit the DUB activity when physiological substrates are used. To circumvent this problem it is possible to run DUB assays with more physiologically related HTS Ub-substrates. An isopeptide linkage reflects the physiological target of most of the DUBs and thus it is expected to obtain more relevant kinetic data ther than using the classical linear Ub reporters. The development of these substrates is a significant improvement within recent years and may boost the identification of better DUB inhibitors in future.
Ub-Lys-TAMRA-Gly
This fluorescent polarisation reagent consists of a TAMRA-linked peptide that is attached to the C-terminal end of Ub. Importantly, hereby the linkage between the fluorophore and Ub mimics an isopeptide bond between Ub and the target protein23. This substrate allows continuous fluorescence polarisation measurement after cleavage of the isopeptide bond between ubiquitin and the ε-amino group of a lysine. Therefore, many DUBs that do not effectively catalyse Ub-AMC cleavage show robust catalytic activity in isopeptide-based assays.
DiUb-based HTS substrates
Most of the previously described in vitro methods to determine DUB activity make use of artificial substrates. Despite the fact that those assays are easily amenable to HTS there are limitations, especially regarding studies that focus on linkage specificity of DUBs. Here the DiUb-based probes comprise a big advantage. In DiUb-based probes the ε-amino group of a lysine residue of one Ub is linked to the C-terminus of the other and a FRET-based approach is employed.
Different types of FRET pairs can be used to monitor the cleavage of the DiUb substrate. One such substrate is the internally quenched fluorescence (IQF) substrate with each Ub being labelled with a single molecule; either a fluorescence reporter or a quenching dye (IQF-DiUb™ by Lifesensors Inc.). The combination of a specific fluorescent donor (= reporter) and acceptor pair allows a maximal overlap between the two fluorophores, and the donor emission can be monitored as readout24. The cleavage of the IQF DiUb by DUBs leads to release of the ‘quenching Ub’ and, consequently, the increasing donor emission correlates with the corresponding DUB activity (Figure 1). In the meantime, all linkage types are available to test specificity and to measure kinetics of a variety of DUBs.
Activity-based probes
Classic activity-based probes (ABP) for DUBs are based on a single ubiquitin and C-terminally flanked by a warhead such as vinyl sulfone (VS), vinylmethyl ester (VMA) or propargylmide (PA). These warheads are designed to trap the active site of DUBs in an in vitro setting, but also in cell-based systems. To allow detection, most ABPs can be epitope-tagged at the N-terminus of the Ub with various tags, such as HA, biotin or fluorescent dyes (e.g., TAMRA). ABPs have been successfully used to screen for specificity of small-molecule DUB inhibitors in cells. This method allows for the cell-based profiling of DUB inhibitors by mass spectrometry (MS)25. However, so far these DUB-specific ABPs are not designed for high-throughput settings as most of the readouts are SDS-PAGE- or MS-based and do not address the ubiquitin chain linkage specificity that certain DUBs possess26.
With regard to this issue, a new class of di-ubiquitin based probes represents a major advantage: diUb based probes mimic more physiological conditions, even though the Michael’s acceptor – which binds the catalytic cysteine – is introduced between the distal and proximal ubiquitin27. To finally be able to use these methods for high-throughput DUB inhibitor screening, Ub or diUb-ABPs that are coupled to a fluorophore could be investigated using fluorescence polarisation — a robust method to study molecular interactions28. FluoPol-ABP assays have been shown to be of great value in the identification of small molecule enzyme inhibitors for various enzyme classes and these can be HTS-applicable, as they are adjustable to the 384 well plate format29 (Figure 1).
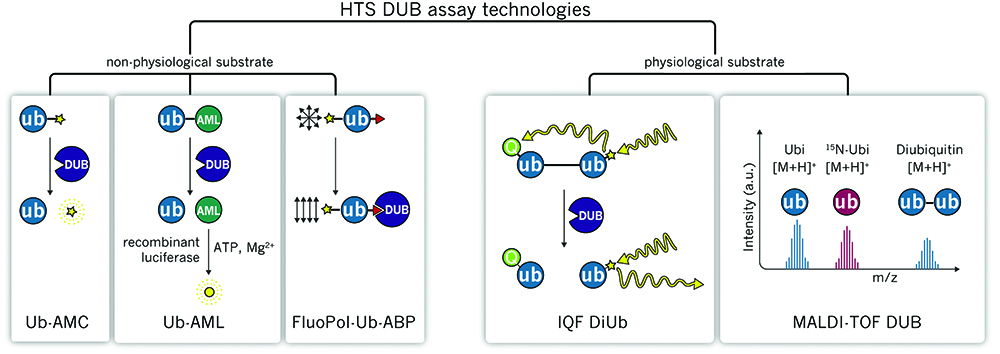
Figure 1: Graphical illustration of non-physiological and physiological DUB assays
MALDI-TOF DUB
Ritorto et al. have developed a HTS-compatible in vitro assay termed MALDI-TOF DUB to assess enzyme activity using physiological substrates30. All different Ub linkages can be tested using recombinant DiUb and recombinantly purified proteins, which are subjected to a low volume DUB assay in a microtiter plate. Following termination of the reaction and sample preparation, the mixture is spotted on a MTP AnchorChip 1536 TF and analysed by matrix-assisted laser desorption/ionisation time-of-flight (MALDI-TOF) MS. 15N-labelled Mono-ubiquitin serves as an internal control in each sample, which allows the calculation of the ratio of (cleaved) ubiquitin/15N-ubiuqitin to uncleaved di-ubiquitin to be calculated (Figure 1).
A sensitive and fast mass spectrometer (ultraflextreme MALDI-TOF, Bruker Daltonics) together with the utilised MTP AnchorChips makes the analysis of 1,536 samples possible in fewer than three hours. This extremely fast readout has great advantages over the standard low-throughput in vitro DUB assay followed by SDS-PAGE18. Importantly, in this assay, linkage specificity and small molecule activity is analysed together. Furthermore, this method requires only small amounts of substrate and enzyme (low nanomolar range) and is partially adapted to automation. Taken together, this method can be a powerful and fast tool to analyse enzyme activity in HTS settings.
Conclusion
Identification of potent and selective inhibitors for drug discovery relies largely on particular assays used during the screening campaign and at the validation stage. This is of critical importance, since screening of large libraries may generate false positive hits and these need to be eliminated during hit validation steps by conducting confirmation as well as counter assays.
DUBs are proteases with specialised functions and recent high/medium-throughput technologies have provided novel opportunities to target distinct DUBs for drug discovery purposes. Most of the assays discussed in this report can be used at the HTS stage and are suitable for the verification step after first screening hits have been identified. Importantly, some of these assay types can also be applied to other closely related proteases by simply using different substrates. This is particularly useful to triage compounds towards specificity and selectivity. Taken together, having these tools in hand researchers can now pursue finding novel first-in-class drugs for the DUB target class.
Biographies
KENJI SCHORPP is Senior Staff Scientist at the Assay Development and Screening Platform, HelmholtzZentrum München, Munich, Germany. He is responsible for assay development and screening of early drug discovery projects and has a special interest in targeting the ubiquitin system. Prior to this, he worked as a Postdoc and gained his PhD at the Max-Planck-Institute of Biochemistry where he had already been focusing on ubiquitin and ubiquitin-like proteins.
ELISABETH WEBER is a Student Research Assistant at the Assay Development and Screening Platform, HelmholtzZentrum München, Munich, Germany. Her current research involves targeting protein-protein interactions in the ubiquitin system. Prior to this, she completed her Bachelor Thesis at the Assay Development and Screening Platform, HelmholtzZentrum München and her Master Thesis at the University of California, Berkeley, both times focusing on ubiquitin-related signalling. Further steps in her early career include research in the Reagents and Assay Development Unit at AstraZeneca, Mölndal, Sweden.
KAMYAR HADIAN is the Head of the Assay Development and Screening Platform at HelmholtzZentrum München, Munich, Germany. His research focus is the identification of molecular mediators of ubiquitin signalling and the development of strategies to interfere with these novel targets. Moreover, he has in-depth experience in biochemical and cell-based assays for high-throughput and high-content screenings. Kamyar studied biology at the Technical University of Munich and received his PhD from the Ludwig-Maximilians-University in 2009 for his studies in HIV research. He did a postdoc in the field of NF-κB signalling, before being appointed to his current role.
References
- Hershko A, Ciechanover A. The ubiquitin system. Annual review of biochemistry. 1998; 67, 425-79
- Chen ZJ, Sun LJ. Nonproteolytic functions of ubiquitin in cell signaling. Molecular cell. 2009; 33 (3), 275-86
- Mizushima N, Noda T, Yoshimori T, et al. A protein conjugation system essential for autophagy. Nature; 1998, 395 (6700), 395-8
- Ichimura Y, Kirisako T, Takao T, et al. A ubiquitin-like system mediates protein lipidation. Nature. 2000; 408 (6811), 488-92
- Reyes-Turcu FE, Ventii KH, Wilkinson KD. Regulation and cellular roles of ubiquitin-specific deubiquitinating enzymes. Annual review of biochemistry. 2009; 78, 363-97
- Heideker J, Wertz IE. DUBs, the regulation of cell identity and disease. The Biochemical Journal. 2015; 467 (1), 191
- Li M, Chen D, Shiloh A, et al. Deubiquitination of p53 by HAUSP is an important pathway for p53 stabilization. Nature 2002, 416 (6881), 648-53
- Kon N, Kobayashi Y, Li M, et al. Inactivation of HAUSP in vivo modulates p53 function. Oncogene 2010, 29 (9), 1270-9
- Reverdy C, Conrath S, Lopez R, et al. Discovery of specific inhibitors of human USP7/HAUSP deubiquitinating enzyme. Chemistry & biology 2012, 19 (4), 467-77
- Fan YH, Cheng J, Vasudevan SA, et al. USP7 inhibitor P22077 inhibits neuroblastoma growth via inducing p53-mediated apoptosis. Cell death & disease. 2013; 4, e867
- Bignell GR, Warren W, Seal S, et al. Identification of the familial cylindromatosis tumour-suppressor gene. Nature Genetics. 2000; 25 (2), 160-5
- Trompouki E, Hatzivassiliou E, Tsichritzis T, et al. CYLD is a deubiquitinating enzyme that negatively regulates NF-kappaB activation by TNFR family members. Nature. 2003; 424 (6950), 793-6
- Kovalenko A, Chable-Bessia C, Cantarella G, et al. The tumour suppressor CYLD negatively regulates NF-kappaB signalling by deubiquitination. Nature. 2003; 424 (6950), 801-5
- Brummelkamp TR, Nijman SM, Dirac AM, et al. Loss of the cylindromatosis tumour suppressor inhibits apoptosis by activating NF-kappaB. Nature. 2003; 424 (6950), 797-801
- Colland F. The therapeutic potential of deubiquitinating enzyme inhibitors. Biochemical Society Transactions. 2010; 38 (Pt 1), 137-43
- Drag M, Salvesen GS. Emerging principles in protease-based drug discovery. Nature reviews. Drug Discovery. 2010; 9 (9), 690-701
- Komander D, Clague MJ, Urbe S. Breaking the chains: structure and function of the deubiquitinases. Nature reviews. Molecular Cell Biology. 2009; 10 (8), 550-63
- Hospenthal MK, Mevissen TE, Komander D. Deubiquitinase-based analysis of ubiquitin chain architecture using Ubiquitin Chain Restriction (UbiCRest). Nature Protocols. 2015; 10 (2), 349-61
- Dang LC, Melandri FD, Stein RL. Kinetic and mechanistic studies on the hydrolysis of ubiquitin C-terminal 7-amido-4-methylcoumarin by deubiquitinating enzymes. Biochemistry. 1998; 37 (7), 1868-79
- Hassiepen U, Eidhoff U, Meder G, et al. A sensitive fluorescence intensity assay for deubiquitinating proteases using ubiquitin-rhodamine110-glycine as substrate. Analytical Biochemistry. 2007; 371 (2), 201-7
- Nicholson B, Leach CA, Goldenberg SJ, et al. Characterization of ubiquitin and ubiquitin-like-protein isopeptidase activities. Protein science: a publication of the Protein Society. 2008; 17 (6), 1035-43
- Orcutt SJ, Wu J, Eddins MJ, et al. Bioluminescence assay platform for selective and sensitive detection of Ub/Ubl proteases. Biochimica et Biophysica Acta. 2012; 1823 (11), 2079-86
- Tirat A, Schilb A, Riou V, et al. Synthesis and characterization of fluorescent ubiquitin derivatives as highly sensitive substrates for the deubiquitinating enzymes UCH-L3 and USP-2. Analytical Biochemistry. 2005; 343 (2), 244-55
- Johansson MK, Cook RM. Intramolecular dimers: a new design strategy for fluorescence-quenched probes. Chemistry. 2003; 9 (15), 3466-71
- Altun M, Kramer HB, Willems LI, et al. Activity-based chemical proteomics accelerates inhibitor development for deubiquitylating enzymes. Chemistry & Biology. 2011; 18 (11), 1401-12
- Kramer HB, Nicholson B, Kessler BM, et al. Detection of ubiquitin-proteasome enzymatic activities in cells: application of activity-based probes to inhibitor development. Biochimica et Biophysica Acta. 2012; 1823 (11), 2029-37
- Li G, Liang Q, Gong P, et al. Activity-based diubiquitin probes for elucidating the linkage specificity of deubiquitinating enzymes. Chemical Communications. 2014; 50 (2), 216-8
- Owicki JC. Fluorescence polarization and anisotropy in high throughput screening: perspectives and primer. Journal of Biomolecular Screening. 2000; 5 (5), 297-306
- Bachovchin DA, Brown SJ, Rosen H, et al. Identification of selective inhibitors of uncharacterized enzymes by high-throughput screening with fluorescent activity-based probes. Nature Biotechnology. 2009; 27 (4), 387-94
- Ritorto MS, Ewan R, Perez-Oliva AB, et al. Screening of DUB activity and specificity by MALDI-TOF mass spectrometry. Nature Communications. 2014; 5, 4763
Related topics
Assays, DNA, Enzymes, High-Throughput Screening (HTS), Screening, Small molecule