Immune checkpoint inhibitors: targets on the horizon
Posted: 19 April 2015 | Kerstin Papenfuss (MRC Technology) | No comments yet
Immune checkpoint inhibitory antibodies have provided a great conceptual change in the field of immune-oncology. Rather than targeting the tumour directly, these novel drugs are aimed at preventing the interactions between the tumour and the immune system, which the cancer exploits to evade immune recognition…
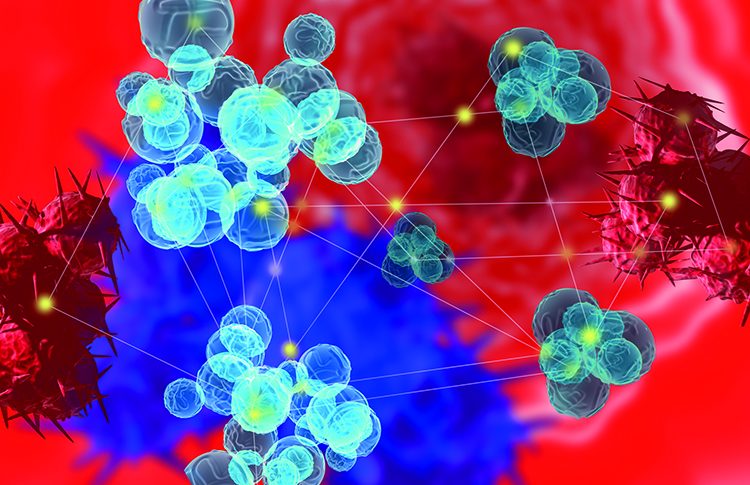
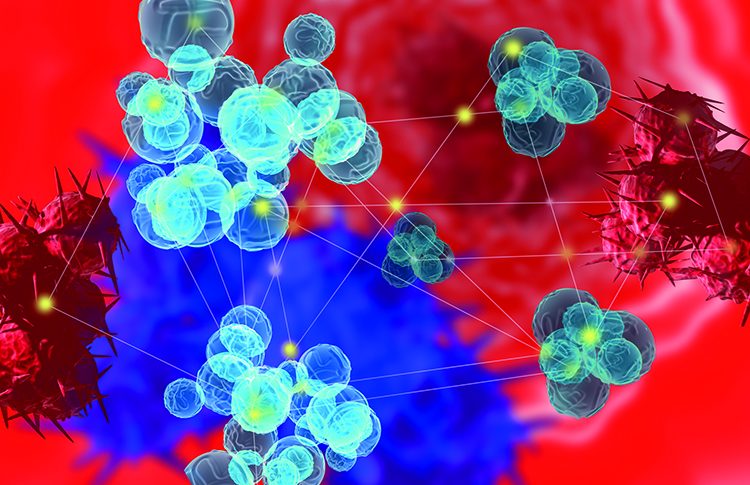
Antibodies against cytotoxic T lymphocyte –associated antigen 4 (CTLA-4) and programmed cell death 1 (PD-1) are now on the market and they have been the trailblazers in this new era of cancer treatment, showing unprecedented success in clinical trials. Their success has led to a growing appetite for novel immune checkpoint inhibitors and this article provides an overview of some up and coming targets in the immune checkpoint inhibitor space.
CTLA-4 and PD-1 antibodies have paved the way for a new therapeutic era in cancer treatment. Classed as immune checkpoint inhibitors, they activate the body’s own immune system to fight the tumour. Due to astonishing results in clinical trials such agents have lately attracted lot of attention, with Prof James P. Allison and Prof. Tasuku Honjo having recently been awarded the first Tang prize in biopharmaceutical science to honour their contributions to the development of CTLA-4 and PD-1 antibodies as a novel approach to activate anti-tumour immunity1.
Cancer cells have developed different mechanisms to actively escape recognition by the immune system. Research over the past decades has improved our understanding of these immune evasion strategies, one of them being the manipulation of T cell activation. Tumours basically hide in plain sight by expressing molecules that shut down T cell activation, thereby preventing the T cells from killing their cells. The new immune checkpoint inhibitors effectively unmask the tumour to the immune system by blocking the interactions between the ligand expressed on the cancer cell and the receptor expressed in the T cell, for example, PD-1L/PD-1 interaction. This leads to sustained activity of the T cells against the tumour cells.
A lot of research has been carried out to unveil the complex interplay of the co-inhibitory and co-stimulatory molecules important for T cell activation and it has become clear that T cell inhibitory receptors represent novel therapeutic targets for chronic infection and cancer. Commercialised antibodies targeting CTLA-4, such as Bristol-Myers Squibb’s ipilimumab (Yervoy), and those targeting PD-1, such as nivolumab (marketed by Ono Pharmaceuticals and Bristol-Myers Squibb) and pembrolizumab (Keytruda; Merck) all show astonishing and unprecedented results in advanced melanoma, a cancer which is notoriously hard to treat2-4. They are now in clinical trials for other types of cancers, where they are also showing promising results. However, not all patients respond to CTLA-4 or PD-1 blockade, some cancer types have proven to be largely refractory to therapy and there is still a lack of biomarkers to predict responsiveness to these treatments.
The successes of the PD-1 and CTLA-4 antibodies, as well as their limitations, have sparked research into alternative immune checkpoint receptor targets. Next in line in clinical development are approaches targeting lymphocyte activation gene-3 (LAG3, CD223). LAG3 was cloned in 1990 and shares 20% sequence identity with the CD4 gene. LAG3 is only expressed on T cells several days after T cell activation5. Hence, blocking LAG3 is less likely to lead to the autoimmune side effects associated with CTLA-4 antibody therapy. LAG3’s role in negative regulation of T cells has been firmly established over the last decades6. In the context of cancer, LAG3 was shown to be upregulated in tumour-infiltrating lymphocytes where it is co-expressed with PD-17. In line with this, blockade of LAG3 in animal models alone has been shown to enhance antitumour T-cell responses8, however combination with PD-1 antibodies was shown to be more efficacious9.
Although the antitumour effects of LAG3 antibodies have largely been attributed the effects they have on LAG3 expressing CD8+ T cells, the action of the antibodies might be more pleiotropic which makes LAG3 particularly attractive as a drug target. MHC Class II, the binding partner of LAG3, is normally expressed on antigen presenting cells (APCs) but has also been shown to be expressed on tumours cell where the LAG3 binding protects from apoptosis10. Hence, LAG3 antibodies could also have a direct effect on tumour cells apoptosis. Furthermore, LAG3 expression has also been shown on regulatory T cells where LAG3 mediates immune suppression in a model for inflammation11; however, it has yet to be investigated whether this is also the case in the context of cancer.
Bristol-Myers Squibb and Ono Pharmaceuticals are co-developing an antagonistic antibody (BMS-986016) which is currently in Phase I clinical trials as a single agent for relapsed or refractory chronic lymphocytic leukemia and lymphomas and as a combination treatment with PD-1 antibody nivolumab for solid tumours. Immutep is pursuing an alternative approach with IMP-312, a soluble LAG3-Ig (4x) fusion which activates major histocompatibility complex (MHC) class II on APCs which is in clinical development for different solid tumours12. IMP-321 was first assessed in a Phase I dose escalation trial for renal cell carcinoma. The trial demonstrated activity at higher doses, which correlated with sustained CD8+T cell activation, and absence of toxicity12. Since then it has been tested in metastatic breast cancer patients in combination with the first-line treatment paclitaxel, showing promising response rates of 50%13.
Targets in earlier-stage discovery
An intriguing preclinical candidate is the immune checkpoint receptor TIM-3 (T cell immunoglobulin and mucin-domain containing-3). TIM-3 was first discovered about a decade ago as a receptor selectively expressed on IFN-γ-producing T cells (CD4+ Th1 cells and CD8+ cytotoxic T cells)14. TIM-3 was first studied in the context of autoimmunity and anti-TIM-3 antibodies showed an exacerbating effect in the EAE (experimental autoimmune encephalomyelitis) model, the most commonly used experimental model for multiple sclerosis (MS). These studies provided the first evidence that TIM-3 might act as a T-cell inhibitory receptor14. Galectin-9 was then identified as the endogenous ligand for TIM-3; in line with this, recombinant Galectin-9 in vivo resulted in selective loss of IFN-γ -producing cells and suppression of Th1 autoimmunity15. These studies collectively indicated a role for TIM-3 as an immune checkpoint receptor.
The first indication of the role of TIM-3 in immune evasion of cancer cells was provided in mouse models of solid and hematologic malignancies, where TIM-3 expression was found on the most dysfunctional and most suppressed CD8+ T cells. The expression of TIM-3 was then confirmed on T cells isolated from cancer patients. Approximately one third of CD8+ cytotoxic T cells in the vicinity of the tumour were found to express TIM-3 in advanced melanoma16, non-small cell lung cancer17 and B-cell lymphoma18. Similarly to LAG3, TIM-3 expression in these three cancers also correlated with PD-1 expression, indicating that blockade of both receptors might be advantageous to prevent conversion of activated T cells into dysfunctional ones. In addition to the expression of TIM-3 on CD8+ T cells, TIM-3 expression on tumour-associated regulatory T cells (Tregs) also seems to contribute to the immunosuppressive effects observed in the tumour environment. In multiple cancer models, TIM-3-positive Treg populations have been shown to be more immunosuppressive than their TIM-3-negative counterparts19.
Immunosuppression in the tumour environment through TIM-3 on CD8+cells and Tregs makes TIM-3 a highly attractive target, as TIM-3 blocking agents could have a dual mode of action to disable tumour immune evasion by reconstituting CD8+ T cell anti-tumour activity and by suppressing TIM-3+ Treg mediated immunosuppression. TIM-3 blockade alone has been shown to be effective in preclinical cancer models from tissues of different origins and shows comparable activity to PD-1 blockade alone20. However, the effect was even greater when combined with PD-1 antibodies, supporting the notion that combinatorial treatment with a PD-1/PD-1L blocking agent may be most effective in a clinical setting. Also the structure of TIM-3 supports the combination with PD-1 blocking approaches: unlike PD-1 and other members of the cell checkpoint receptors, TIM-3 does not have an immunoreceptor tyrosine-based inhibition motif (ITIM) in its intracellular tail meaning there is little potential for functional redundancy between the two.
Blockade of TIM-3 should be associated with lower side effects than, for example, CTLA-4 antibodies, which are associated with autoimmune side effects that are attributed to the constitutive expression of CTLA-4 on effector T cells. In contrast TIM-3 is rather selectively expressed in INF-γ-producing T cells and in T cells in the tumour environment. Hence, TIM-3 antibodies are less likely to cause overall autoimmune effects. In light of all these unique features of TIM-3 as an immune checkpoint receptors, it is not surprising that several companies are pursuing TIM-3 as an antibody target. Programs still appear to be at preclinical stage and some have reported positive results of their lead candidates in preclinical models.
The ligands of CTLA4 and PD-1 belong to the B7 family of costimulatory molecules, so it is not surprising that other members of this family have also been in the focus of research. However, progress has been hampered by the fact that the receptors for some of the remaining ligands in this family (B7-H3, B7-H4, B7-H5) have not yet been identified.
B7 homologue 3 (B7-H3 or CD276) shares a 31% sequence homology with PD-1L, the ligand of PD-1. B7-H3 is constitutively expressed in murine APCs but needs to be induced in human immune cells. Also, its expression is not restricted to immune cells. It seems rather widely expressed in other cells types such as osteoblasts, fibroblasts and epithelial cells. Importantly, high expression of B7-H3 has been reported in breast, pancreatic, prostate, ovarian and renal cell carcinoma. There is some controversy as to whether B7-H3 is co-stimulatory or co-inhibitory21,22. Similarly, B7-H3 expression has also been reported to either correlate with improved prognosis or poor prognosis in cancer. Ectopic expression experiments in murine cancer models largely seem to suggest a co-stimulatory role for B7-H3, as they have shown to induce tumour regression or reduce tumour growth23,24. In contrast to this, experiments involving B7-H3-blocking antibodies have shown anti-tumour activity in xenograft models25. These opposing results suggest that the role of B7-H3 is rather more complex and potentially context-dependent. Macrogenics’ antibody MGA271 is now in early-stage clinical trials for B7-H3-positive metastatic/recurrent adenocarcinoma which will hopefully shed some light on the situation.
B7 homologue 4 (B7-H4, B7S1, B7x, VTcn1) is a more recent addition to the B7 family, having been identified in 2003. It is well documented that B7-H4 inhibits T cell responses26. In healthy individuals, the protein is mainly expressed in APCs whereas the mRNA is detected ubiquitously, indicating translational regulation27. B7-H4 expression has been shown to be upregulated in numerous human cancers and seems to be linked to poor prognosis28. This expression profile makes B7-H4 an attractive target for immune-oncology and results obtained in animal models further support this notion. B7-H4 KO mice show fewer lung metastases compared to wildtype mice in a model for lung metastasis29 and blockade of B7-H4 using an anti-B7-H4 single chain fv was able to delay tumour growth in ovarian cancer xenograft experiments30.
B7 homologue 5 (B7-H5), more commonly referred to as VISTA, has only recently been described to be a negative checkpoint regulator31. It is predominantly expressed on haematopoietic cells and is highly expressed on APCs and T cells. When produced as an Ig fusion protein or overexpressed on APCs, it inhibits CD4+ and CD8+ T cell proliferation and production of cytokines. VISTA negatively regulates CD4+ T cell responses by suppressing early TCR activation and arresting cell division but has a minimum direct impact on apoptosis. VISTA-Ig has been shown to promote the induction of Foxp3+ Tregs in vitro and regulates their suppressive activity. VISTA blocking antibodies showed antitumour activity in mouse models, correlating with an elevated number of tumour specific T cells in the tumour environment32.
Taken together, there are some promising drug targets in the orphan B7 ligand space. Identification of their receptors will be essential for further characterisation of the ligands as drug targets or could potentially reveal novel drug targets. Future research will also revolve around the identification of ideal combinations of checkpoint inhibitors to achieve optimal anti-tumour activity of T cells as well as around the definition of biomarkers to predict responding patient populations.
Biography
References
- Chen YS, Shen CR. Immune checkpoint blockade therapy: The 2014 Tang prize in biopharmaceutical science. Biomedical journal. 2015;38(1):5-8.
- Hodi FS, O’Day SJ, McDermott DF, Weber RW, Sosman JA, Haanen JB, et al. Improved survival with ipilimumab in patients with metastatic melanoma. The New England journal of medicine. 2010;363(8):711-23.
- Hamid O, Robert C, Daud A, Hodi FS, Hwu WJ, Kefford R, et al. Safety and tumor responses with lambrolizumab (anti-PD-1) in melanoma. The New England journal of medicine. 2013;369(2):134-44.
- Topalian SL, Sznol M, McDermott DF, Kluger HM, Carvajal RD, Sharfman WH, et al. Survival, durable tumor remission, and long-term safety in patients with advanced melanoma receiving nivolumab. Journal of clinical oncology : official journal of the American Society of Clinical Oncology. 2014;32(10):1020-30.
- Workman CJ, Rice DS, Dugger KJ, Kurschner C, Vignali DA. Phenotypic analysis of the murine CD4-related glycoprotein, CD223 (LAG-3). European journal of immunology. 2002;32(8):2255-63.
- Sierro S, Romero P, Speiser DE. The CD4-like molecule LAG-3, biology and therapeutic applications. Expert opinion on therapeutic targets. 2011;15(1):91-101.
- Demeure CE, Wolfers J, Martin-Garcia N, Gaulard P, Triebel F. T Lymphocytes infiltrating various tumour types express the MHC class II ligand lymphocyte activation gene-3 (LAG-3): role of LAG-3/MHC class II interactions in cell-cell contacts. European journal of cancer. 2001;37(13):1709-18.
- Grosso JF, Kelleher CC, Harris TJ, Maris CH, Hipkiss EL, De Marzo A, et al. LAG-3 regulates CD8+ T cell accumulation and effector function in murine self- and tumor-tolerance systems. The Journal of clinical investigation. 2007;117(11):3383-92.
- Woo SR, Turnis ME, Goldberg MV, Bankoti J, Selby M, Nirschl CJ, et al. Immune inhibitory molecules LAG-3 and PD-1 synergistically regulate T-cell function to promote tumoral immune escape. Cancer research. 2012;72(4):917-27.
- Hemon P, Jean-Louis F, Ramgolam K, Brignone C, Viguier M, Bachelez H, et al. MHC class II engagement by its ligand LAG-3 (CD223) contributes to melanoma resistance to apoptosis. Journal of immunology. 2011;186(9):5173-83.
- Huang CT, Workman CJ, Flies D, Pan X, Marson AL, Zhou G, et al. Role of LAG-3 in regulatory T cells. Immunity. 2004;21(4):503-13.
- Brignone C, Escudier B, Grygar C, Marcu M, Triebel F. A phase I pharmacokinetic and biological correlative study of IMP321, a novel MHC class II agonist, in patients with advanced renal cell carcinoma. Clinical cancer research : an official journal of the American Association for Cancer Research. 2009;15(19):6225-31.
- Brignone C, Gutierrez M, Mefti F, Brain E, Jarcau R, Cvitkovic F, et al. First-line chemoimmunotherapy in metastatic breast carcinoma: combination of paclitaxel and IMP321 (LAG-3Ig) enhances immune responses and antitumor activity. Journal of translational medicine. 2010;8:71.
- Monney L, Sabatos CA, Gaglia JL, Ryu A, Waldner H, Chernova T, et al. Th1-specific cell surface protein Tim-3 regulates macrophage activation and severity of an autoimmune disease. Nature. 2002;415(6871):536-41.15. Zhu C, Anderson AC, Schubart A, Xiong H, Imitola J, Khoury SJ, et al. The Tim-3 ligand galectin-9 negatively regulates T helper type 1 immunity. Nature immunology. 2005;6(12):1245-52.
- Fourcade J, Sun Z, Benallaoua M, Guillaume P, Luescher IF, Sander C, et al. Upregulation of Tim-3 and PD-1 expression is associated with tumor antigen-specific CD8+ T cell dysfunction in melanoma patients. The Journal of experimental medicine. 2010;207(10):2175-86.
- Gao X, Zhu Y, Li G, Huang H, Zhang G, Wang F, et al. TIM-3 expression characterizes regulatory T cells in tumor tissues and is associated with lung cancer progression. PloS one. 2012;7(2):e30676.
- Yang ZZ, Grote DM, Ziesmer SC, Niki T, Hirashima M, Novak AJ, et al. IL-12 upregulates TIM-3 expression and induces T cell exhaustion in patients with follicular B cell non-Hodgkin lymphoma. The Journal of clinical investigation. 2012;122(4):1271-82.
- Sakuishi K, Ngiow SF, Sullivan JM, Teng MW, Kuchroo VK, Smyth MJ, et al. TIM3FOXP3 regulatory T cells are tissue-specific promoters of T-cell dysfunction in cancer. Oncoimmunology. 2013;2(4):e23849.
- Anderson AC. Tim-3: an emerging target in the cancer immunotherapy landscape. Cancer immunology research. 2014;2(5):393-8.
- Chapoval AI, Ni J, Lau JS, Wilcox RA, Flies DB, Liu D, et al. B7-H3: a costimulatory molecule for T cell activation and IFN-gamma production. Nature immunology. 2001;2(3):269-74.
- Suh WK, Gajewska BU, Okada H, Gronski MA, Bertram EM, Dawicki W, et al. The B7 family member B7-H3 preferentially down-regulates T helper type 1-mediated immune responses. Nature immunology. 2003;4(9):899-906.
- Luo L, Chapoval AI, Flies DB, Zhu G, Hirano F, Wang S, et al. B7-H3 enhances tumor immunity in vivo by costimulating rapid clonal expansion of antigen-specific CD8+ cytolytic T cells. Journal of immunology. 2004;173(9):5445-50.
- Sun X, Vale M, Leung E, Kanwar JR, Gupta R, Krissansen GW. Mouse B7-H3 induces antitumor immunity. Gene therapy. 2003;10(20):1728-34.
- Loo D, Alderson RF, Chen FZ, Huang L, Zhang W, Gorlatov S, et al. Development of an Fc-enhanced anti-B7-H3 monoclonal antibody with potent antitumor activity. Clinical cancer research : an official journal of the American Association for Cancer Research. 2012;18(14):3834-45.
- Sica GL, Choi IH, Zhu G, Tamada K, Wang SD, Tamura H, et al. B7-H4, a molecule of the B7 family, negatively regulates T cell immunity. Immunity. 2003;18(6):849-61.
- Choi IH, Zhu G, Sica GL, Strome SE, Cheville JC, Lau JS, et al. Genomic organization and expression analysis of B7-H4, an immune inhibitory molecule of the B7 family. Journal of immunology. 2003;171(9):4650-4.
- He C, Qiao H, Jiang H, Sun X. The inhibitory role of b7-h4 in antitumor immunity: association with cancer progression and survival. Clinical & developmental immunology. 2011;2011:695834.
- Abadi YM, Jeon H, Ohaegbulam KC, Scandiuzzi L, Ghosh K, Hofmeyer KA, et al. Host b7x promotes pulmonary metastasis of breast cancer. Journal of immunology. 2013;190(7):3806-14.
- Dangaj D, Lanitis E, Zhao A, Joshi S, Cheng Y, Sandaltzopoulos R, et al. Novel recombinant human b7-h4 antibodies overcome tumoral immune escape to potentiate T-cell antitumor responses. Cancer research. 2013;73(15):4820-9.
- Wang L, Rubinstein R, Lines JL, Wasiuk A, Ahonen C, Guo Y, et al. VISTA, a novel mouse Ig superfamily ligand that negatively regulates T cell responses. The Journal of experimental medicine. 2011;208(3):577-92.
- Le Mercier I, Chen W, Lines JL, Day M, Li J, Sergent P, et al. VISTA Regulates the Development of Protective Antitumor Immunity. Cancer research. 2014;74(7):1933-44.
Related topics
Antibodies, Cytokines, Immuno-oncology, Oncology, T cells, Therapeutics