Synthetic biology in drug discovery
Posted: 13 September 2018 | Dr Tim Brears - Chief Executive Officer - Evonetix | No comments yet
Synthetic biology is broadly defined as the design and construction of novel artificial biological pathways, organisms or devices; or the redesign of existing natural biological systems.1 It brings together a range of disciplines and skills, from biology and chemistry to computing, bioinformatics and engineering.
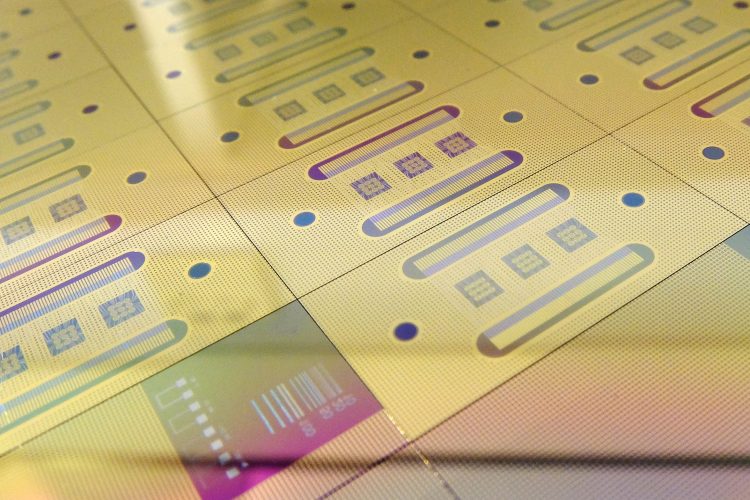
RMG Photography - November 2017 Evonetix - Chesterford Research Park. Pic - Richard Marsham/RMG Photography RMG Photography - Tel : 07798 758711 www.rmg-photography.co.uk
It is generally accepted that synthetic biology is poised to deliver a wide range of new products and to disrupt a number of industries including pharmaceuticals, agriculture, renewables and industrial biotech. It presents the opportunity to exploit biology as never before and could be fundamental in helping us manage the Earth’s resources. However, to achieve its full potential, synthetic biology will require the continued development and convergence of its underlying skills and disciplines, to enable the rational and predictable design of biological systems.
The history of pharmaceutical drugs is one of constant development: from the use of natural products to the development of synthetic chemistry as a source of new ingredients and products, the use of antibodies and other biologics, to the emerging potential of gene therapy and personalised medicine.
With the advent of synthetic biology, the ability to explore, select and optimise the biology around us is greatly enhanced. This has led to much anticipation of significant and broad impact in the drug discovery and development field, building on some notable successes already achieved.
Drug screening
Drug discovery has historically been led by the screening of small-molecule chemical libraries against targets suitable for therapeutic intervention.
In drug screening, the tools of molecular biology, used more efficiently as enabled by synthetic biology, will facilitate many opportunities. In cell-based assays, these include improving the expression of the screening target and developing more efficient assay systems by manipulating reporter genes and the pathways that result in the expression of reporters.
Synthetic biology also offers the ability to generate large libraries of variation and therefore the prospect of creating and selecting novel activities. Polyketides offer an interesting example. They represent an important class of natural products and include, for example, the antibiotic erythromycin, the anti-cancer agent epothilone and the immunosuppressant FK-506. Polyketides are built in a modular fashion by polyketide synthases, whose component parts can be separated and recombined to generate novel enzymes that synthesise novel polyketides. By recombining enzyme modules, libraries of polyketides with novel biological activities can be generated and screened for useful properties.
Similarly, it is possible to generate libraries of cyclic peptides, which include well-known antibiotic (vancomycin), immunosuppressant (cyclosporin) and anti-cancer (actinomycin) examples. Although it is possible to make libraries of cyclic peptides chemically, genetically-encoded libraries have several advantages as they are more readily accessible and allow straightforward hit deconvolution.2
Protein engineering and the design of novel therapeutics
The power of synthetic biology is clearly demonstrated by its ability to engineer novel proteins for use as therapeutics. It is this rational design of many variants, guided by protein structural expertise, that enables the directed evolution of therapeutic proteins with desirable properties, including gastrointestinal (GI) stability and immune tolerance.
Leading protein engineers have successfully used this approach to generate an active, GI-stable, orally administered, therapeutic enzyme candidate to treat phenylketonuria (PKU).3 PKU is a rare, autosomal recessive genetic disorder caused by a mutation in the gene encoding the hepatic enzyme phenylalanine hydroxylase (PAH), which prevents the conversion of the essential amino acid phenylalanine into tyrosine. Without functional PAH, high levels of phenylalanine accumulate in the body and cause serious neurological complications, including intellectual disability, seizures, mental illness, tremors and cognitive and behavioural problems. The approach will allow the oral administration of PKU designed to resist proteolytic attack in the GI tract and compensate for the absence of the missing natural enzyme by hydroxylating phenylalanine.
Using a similar approach, scientists at the University of Washington and PvP Biologics4 have sought to generate an entirely novel protein that degrades gluten, the substance that underlies coeliac disease. Coeliac disease is characterised by an inflammatory intestinal immune response to gluten, a protein found in food containing wheat, rye, and barley. The disease is a serious health problem and results from the inability of human digestive enzymes to completely break down gliadin, the major protein component of gluten because gliadin is highly enriched in the difficult-to-digest amino acids proline and glutamine. Using computational design, the team developed KumaMax, a recombinant enzyme that degrades gluten under acidic stomach conditions and has high specificity for the parts of gluten that trigger the autoimmune reaction leading to coeliac disease. For coeliac patients attempting to maintain a gluten-free diet but who may accidentally ingest gluten, KumaMax has the potential to degrade the immune-reactive parts of gluten before they exit the stomach in order to decrease the immune response to gluten and reduce the symptoms and intestinal damage associated with coeliac disease.
Making biologically-active substances more efficiently – biosynthesis of pharmaceuticals
Recombinant DNA technology has long been used to synthesise biologically-active compounds in novel hosts, but the advancement of synthetic biology offers the prospect of undertaking heterologous production of natural products on a scale not previously contemplated.
An excellent example is provided by the anti-malarial drug artemisinin, a terpenoid naturally produced by the sweet wormwood tree (Artemisia annua), which is difficult, expensive and time-consuming to cultivate. In 2013, Paddon, et al. working at Amyris, reported the development of strains of Saccharomyces cerevisiae engineered with the entire biosynthetic pathway for artemisinic acid,5 a precursor of artemisinin. Production in genetically-modified yeast is cheaper and faster and allows significantly more widespread use of Artemisinin-based Combination Therapies (ACTs), recommended by the World Health Organization as the primary first-line treatment for malaria.6
A further example of a plant-derived medicinal compound is presented by the opioid painkiller hydrocodone and was published by Galanie, et al. of Stanford University and Antheia.7 Opium poppies remain the sole source of opioid medicines,8 but their cultivation is challenging and unpredictable. The Stanford group engineered yeast to produce hydrocodone from sugar, combining enzyme discovery, enzyme engineering, pathway and strain optimisation to realise full opiate biosynthesis in yeast. The genetically-modified hydrocodone-synthesising strains required the expression of 23 enzyme activities from plants, mammals, bacteria and yeast itself.
It is clear that synthetic biology has great potential for the heterologous production of natural products. Starting with pathways known in specific organisms, re-cloning, codon optimisation (for the new host) and generation of gene variants to optimise activity become a reality with access to synthetic DNA.
Novelty in biology
However, the opportunity goes beyond this. The expanding body of sequence information is uncovering a universe of hitherto-unknown opportunities. For example, the wealth of natural products found in GI-inhabiting microbes (including many new antibiotics) must surely represent a trove of achievable opportunities as we develop more effective skills to identify, analyse and optimise the expression of the underlying genes in preferred hosts.
Moreover, as sequence information and the power of bioinformatics increases, we can achieve a better understanding of metabolic pathways, how they might cross-interact and how we might use components from different organisms to create new and interesting target molecules. The tools that will allow us to achieve this will be DNA sequence information, metagenomics, bioinformatics and the ability to synthesise DNA and test many variants.
Other opportunities
There are many other areas where synthetic biology techniques may impact drug discovery. These range from vaccines (antigen genes or nucleic acid vaccines); gene therapy (with its requirement for synthetic DNA), CRISPR-Cas9 and other cellular engineering techniques; the use of non-natural amino acids and their engineering into therapeutic peptides; and ‘DNA origami’ as a means of drug delivery.
What are the challenges?
Synthetic biology is enabled by the vast amounts of DNA sequence information available from a huge array of organisms, and the availability of genomic and metagenomic tools to understand the potential functionality of such information.
It is clear that synthetic biology has the potential to impact many aspects of drug discovery, but the scale of its impact will depend on the further development of the technologies that underlie synthetic biology. These include DNA synthesis, powerful bioinformatics, suitable chassis organisms and the ability to build/engineer pathways and organisms in a predictable fashion.
Crucial for the achievement of many of the opportunities in synthetic biology is the further development of synthetic DNA synthesis. In the same way that DNA sequencing underwent a revolution in the mid-2000s resulting in massive parallelisation and reduction in cost, a comparable breakthrough in DNA synthesis would catalyse many opportunities in this area.
While companies have made great progress in upscaling DNA synthesis, there remain challenges in achieving further scale, greater accuracy and reduced cost. New approaches include the development of ever-smaller sites of synthesis and enzymatic techniques in which base additions are made by template-independent enzymes, such as terminal deoxynucleotidyl transferase (TdT), rather than phosphoramidites. As such, several companies are pursuing enzymatic approaches, with distinct strategies for nucleotide blocking.
Activated silicon, an approach being pioneered by Evonetix, represents an exciting opportunity to achieve highly parallel, high-fidelity DNA synthesis in an environment without the usual physical separation of reaction sites. Independent thermal conditions at each site are used to localise the reactions and maintain exquisite control of de novo DNA synthesis and DNA assembly.
Overcoming some of the challenges to allow robust, scalable and cost-effective synthesis of high-fidelity DNA will enable DNA synthesis to enjoy a sea change similar to sequencing in the mid-2000s.
Biography
TIM BREARS is the Chief Executive of Evonetix Ltd. He has previously served as Chief Executive of a number of bioscience companies that have specialised in the discovery and development of ion-channel-modulating drugs for the control of atrial fibrillation, as well as the development of zinc finger proteins for gene regulation. Tim has spent ten years in the US, initially as a long-term fellow of the European Molecular Biology Organisation at Rockefeller University, New York, and subsequently as Director of Licensing at Ciba-Geigy (later Novartis) Agribusiness in Research Triangle Park, North Carolina. Tim is a graduate of Oxford University, holds a PhD in molecular biology from Cambridge University and an MBA from Duke University’s Fuqua School of Business.
References
- The Royal Society. Synthetic Biology: Project details. [Online] https://royalsociety.org/topics-policy/projects/synthetic-biology/. Accessed July 2018.
- Tavassoli A. SICLOPPS cyclic peptide libraries in drug discovery. Current Opinion in Chemical Biology. 2017; 38:30-35. doi: 10.1016/j.cbpa.2017.02.016.
- Codexis. Biotherapeutics. [Online] https://codexis.com/industries/biotherapeutics/. Accessed July 2018.
- PvP Biologics. [Online] https://www.pvpbio.com/. Accessed July 2018.
- Paddon CJ, Westfall PJ, Benjamin K, et al. High-level semi-synthetic production of the potent antimalarial artemisinin. Nature. 2013; 496: 528-532. doi:10.1038/nature12051.
- Amyris. [Online] https://amyris.com/. Accessed July 2018.
- Antheia Inc. [Online] http://www.antheia.bio/. Accessed July 2018.
- Galanie S, Thodey K, Trenchard IJ, et al. Complete biosynthesis of opioids in yeast. Science. 6252, 2015; 349(6252): 1095-1100. doi:10.1126/science.aac9373.
The rest of this article is restricted - login or subscribe free to access
Thank you for visiting our website. To access this content in full you'll need to login. It's completely free to subscribe, and in less than a minute you can continue reading. If you've already subscribed, great - just login.
Why subscribe? Join our growing community of thousands of industry professionals and gain access to:
- quarterly issues in print and/or digital format
- case studies, whitepapers, webinars and industry-leading content
- breaking news and features
- our extensive online archive of thousands of articles and years of past issues
- ...And it's all free!
Click here to Subscribe today Login here
Related topics
Drug Development, Drug Discovery, Drug Discovery Processes, Drug Targets, Hit-to-Lead, Immunology, Molecular Targets, Technology, Therapeutics
Related organisations
Evonetix