Using droplet digital PCR to monitor the safety of CAR T cells
Posted: 12 December 2019 | Dr Ping Jin (Center for Cellular Engineering at National Institutes of Health Clinical Center) | No comments yet
CAR T-cell therapy has caused quite a stir in the immunotherapy world, but it is a process that requires precision and care. Quantitative polymerase chain reaction (qPCR) has thus far been the stalwart technique for providing a check on copy numbers, but here Ping Jin explains why droplet digital PCR (ddPCR) is more than a match for this technique.
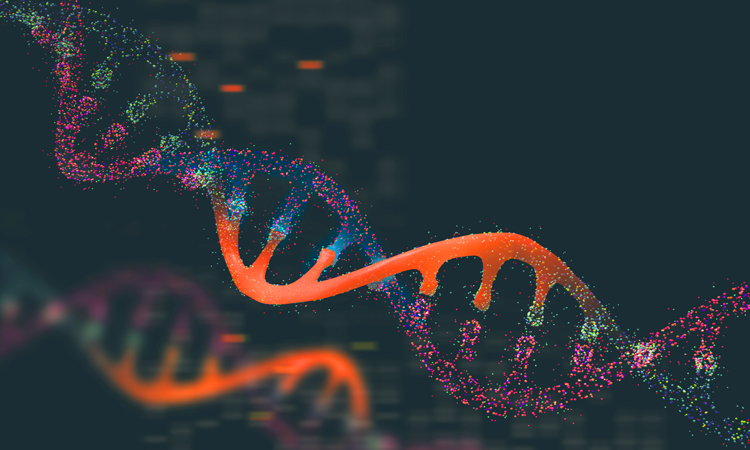
Chimeric antigen receptor (CAR) T cells, first tested in cancer patients less than a decade ago,1 represent the latest generation of adoptive cell therapy and the most personalised approach to date. CAR T-cell therapy is a method of supercharging a patient’s immune system to fight their cancer and it involves three stages. First, T cells are isolated from a patient’s blood; then, using either viral vectors, transposon systems or mRNA transduction,2 T cells are genetically modified to express the CAR protein on their surface. Finally, the T cells are amplified in culture and infused back into the patient where they attack the cancer cells.
CAR T-cell therapy is rapidly gaining popularity as a specific method for treating cancer. According to clinicaltrials.gov, there are currently over 300 active or recruiting CAR T-cell therapy trials underway,3 mostly taking place in the US and China.4 According to one forecast, the global market for CAR T-cell therapies is expected to grow to $8 billion by 2028.5 However, since CAR T cells are a living therapy, as opposed to a synthetic drug, the process of preparing them to assure safety and effectiveness in patients is complex.
Making safe and effective CAR T cells
Integration of the CAR gene into the T-cell genome is a random process that must be closely monitored. If the transgene is inserted into the wrong place, it might not perform optimally, or worse, could potentially cause new cancerous growths to form. For example, a CAR gene could integrate into and activate an oncogene, promoting the growth of T-cell neoplasms. It could also integrate into a non-coding DNA region and lie dormant.
Manufacturers must also monitor transgene copy number because they cannot strictly control how many copies of the CAR gene will integrate into each cell. The World Health Organization’s (WHO) standards for lentiviral vectors in cell therapy states that between one and four copies should integrate into each cell.6 More than four copies per cell exposes patients to toxicity effects: an excess of CARs can lead to an inflammatory response that can cause organ dysfunction and death.7 It is therefore critical that manufacturers record the copy number and location of transgene integrations in CAR T-cell development.
Unlike qPCR, ddPCR directly quantifies target nucleic acids, thus removing the need for a standard curve”
The gold standard method for monitoring integration copy number during CAR T-cell development is qPCR, but this technique requires serial dilutions to generate a standard curve, which is time consuming, technically complex and laborious. Also, qPCR is not sensitive enough to detect integrations of one copy per cell. Rather, the limit of detection for qPCR is approximately two or three copies per cell.
An alternative method for monitoring CAR T-cell integrations that can overcome the limitations of qPCR is ddPCR. qPCR and ddPCR employ similar chemistry: they both amplify target nucleic acid strands using standard PCR reagents, primers and probes. Unlike qPCR, however, ddPCR partitions samples into nanolitre-sized droplets and separate PCR reactions take place in each one. A fluorescent probe binds to the target DNA and, when the target DNA molecule is amplified, it fluoresces. The droplets that do not contain the target molecule do not amplify and therefore do not fluoresce. By counting the number of ‘positive’ versus ‘negative’ droplets it is possible to determine the concentration of the target DNA strand in the sample and, in turn, the average copy number of that target sequence.
Unlike qPCR, ddPCR directly quantifies target nucleic acids, thus removing the need for a standard curve. Also, ddPCR is more sensitive than qPCR and can detect down to one copy per cell.
At the National Institutes of Health (NIH) we manufacture CAR T cells and we recently demonstrated that ddPCR can effectively quantify CAR copy numbers in transfected T cells. First, we used either lentiviral or retroviral vectors to deliver the CAR gene before culturing the cells for 7-21 days. Before infusing them back into the patients, we examined them using flow cytometry for transduction efficiency and ddPCR for integration copy number.
The data showed that ddPCR reliably measured copy number across different technicians, labs and days. Importantly, the results were the same whether the CAR T cells were tested immediately or frozen for three or six weeks.
From the results of previous research, we know transfection efficiency with lentiviruses can vary from 80 down to 30 percent8 and an efficient transfection step is critical to the integration of CAR genes into T cells. After confirming that our transfection process was efficient, we also used ddPCR to examine the effect of multiplicity of infection (MOI) – the ratio of vectors to T cells – as well as the effect of centrifuging the mixture on copy number.
We found that MOI correlated with transfection efficiency and copy number and that these two factors also correlated with each other. Additionally, our ddPCR analysis showed that centrifuging the mixture helped ameliorate the decreased transfection efficiency and target copy number per cell at a lower MOI.
Although transgenes integrate in random locations, they have preferences for certain sites. We therefore used next-generation sequencing (NGS) to identify these preferred sites and mapped the integrations. Our aim is to design a ddPCR probe that targets the transgene, which will enable its selective amplification and allow us to track these integrations in the engineered T cells.
From our data, ddPCR can be used to reliably observe transgene copy number in clinical CAR T products across different time points and between different labs and technicians. ddPCR is a stable and precise method for measuring copy number; it can enhance quality control for CAR T-cell manufacturing and enable further research into the impact of transgene copy number on the safety and efficacy of engineered cellular products.
The future of ddPCR in CAR T quality control
Quantifying CAR transgene integrations is just one potential application for ddPCR in monitoring the safety of CAR T therapy. ddPCR can also be used to detect replication-competent viruses, which, along with integrations into oncogenes, is a concern of the US Food and Drug Administration (FDA) as a potential risk of CAR T-cell therapy. Although only witnessed in animals, replication-competent T cells could theoretically produce rapidly-progressing T-cell neoplasms. The FDA recommends testing clinical vector lots, manufactured cell products and patients after they have been infused for these viruses. ddPCR could potentially be used for this testing.9
“ddPCR can be used to reliably observe transgene copy number in clinical CAR T products across different time points and between different labs and technicians”
In addition, ddPCR can detect microbial contamination, which poses a risk of infection in patients. It could also help monitor MOI and help achieve optimal transfection efficiency.
Finally, ddPCR could be used to measure CAR T persistence. It is not desirable for CAR T cells to survive in the body for longer than a few months, owing to their potential to induce more mutagenesis. ddPCR could be used to monitor the presence of residual CAR T cells in the blood of patients who have received the treatment.
Our data demonstrated application of ddPCR in one aspect of T-cell manufacturing: ensuring that the CAR gene copy number is not high enough to cause toxicity. We believe that ddPCR can one day help us manufacture T cells that are safer and more effective in patients, enabling us to offer a new generation of cancer therapies that brings hope to more patients.
About the author
Dr Ping Jin leads the Product Assurance and Characterization Testing (PACT) section in the Center for Cellular Engineering, Department of Transfusion Medicine (DTM), National Institutes of Health Clinical Center. PACT provides in-process and lot release testing to support the clinical cell processing laboratory, including flow cytometry, automated cell counts, manual cell counts, CFU assays, endotoxin assays and molecular assays to detect recombinant retroviral and lentiviral vectors.
References
- Schultz L, Mackall C. Driving CAR T cell translation forward. Science Translational Medicine. 2019;11(481):eaaw2127.
- Li Y, Huo Y, Yu L, Wang J. Quality Control and Nonclinical Research on CAR-T Cell Products: General Principles and Key Issues. Engineering. 2019;5(1):122-131.
- Search of: “CAR T cell” | Recruiting, Not yet recruiting, Active, not recruiting, Enrolling by invitation Studies – List Results – ClinicalTrials.gov [Internet]. Clinicaltrials.gov. 2019 [cited 7 August 2019]. Available from: https://clinicaltrials.gov/ct2/results?term=%22CAR+T cell%22&Search=Apply&recrs=b&recrs=a&recrs=f&recrs=d&age_v=&gndr=&type=&rslt=
- Search of: “CAR T-Cell” | Recruiting, Not yet recruiting, Active, not recruiting, Enrolling by invitation Studies – Results on Map – ClinicalTrials.gov [Internet]. Clinicaltrials.gov. 2019 [cited 7 August 2019]. Available from: https://clinicaltrials.gov/ct2/results/map/click?map.x=747&map.y=504&term=%22CAR+T-Cell%22&recrs=abdf&mapw=1913
- Insights C. Global CAR-T Cell Therapy Market to be Worth US$ 8 Billion by 2028 – Coherent Market Insights [Internet]. GlobeNewswire News Room. 2019 [cited 7 August 2019]. Available from: https://www.globenewswire.com/news-release/2018/05/31/1514897/0/en/Global-CAR-T-Cell-Therapy-Market-to-be-Worth-US-8-Billion-by-2028-Coherent-Market-Insights.html
- Zhao Y, Stepto H, Schneider C. Development of the First World Health Organization Lentiviral Vector Standard: Toward the Production Control and Standardization of Lentivirus-Based Gene Therapy Products. Human Gene Therapy Methods. 2017;28(4):205-214.
- Brudno J, Kochenderfer J. Toxicities of chimeric antigen receptor T cells: recognition and management. Blood. 2016;127(26):3321-3330.
- Zhang Z, Qiu S, Zhang X, Chen W. Optimized DNA electroporation for primary human T cell engineering. BMC Biotechnology. 2018;18(1).
- Marcucci K, Jadlowsky J, Hwang W, Suhoski-Davis M, Gonzalez V, Kulikovskaya I et al. Retroviral and Lentiviral Safety Analysis of Gene-Modified T Cell Products and Infused HIV and Oncology Patients. Molecular Therapy. 2018;26(1):269-279.
Related topics
Chimeric Antigen Receptors (CARs), Gene Therapy, Immuno-oncology, Immuno-oncology therapeutics, Immunotherapy, Real-time polymerase chain reaction (qPCR), T cells
Related conditions
Cancer