Current state of NAFLD treatments and the promise of in vitro platforms towards better screening of drug candidates
Posted: 4 June 2020 | Aslihan Gokaltun (Harvard Medical School and Shriners Hospitals for Children), Beyza Bulutoglu (Harvard Medical School and Shriners Hospitals for Children), Camilo Rey-Bedón (Harvard Medical School and Shriners Hospitals for Children), Martin L Yarmush (Harvard Medical School and Shriners Hospitals for Children), Osman Berk Usta (Harvard Medical School and Shriners Hospitals for Children), Peony Banik (Harvard Medical School and Shriners Hospitals for Children) | No comments yet
The heterogeneous pathogenesis of metabolic fatty liver diseases presents researchers with numerous challenges when trying to develop a treatment. This article explores the spectrum of these diseases and presents a novel in vitro platform for screening drug candidates.
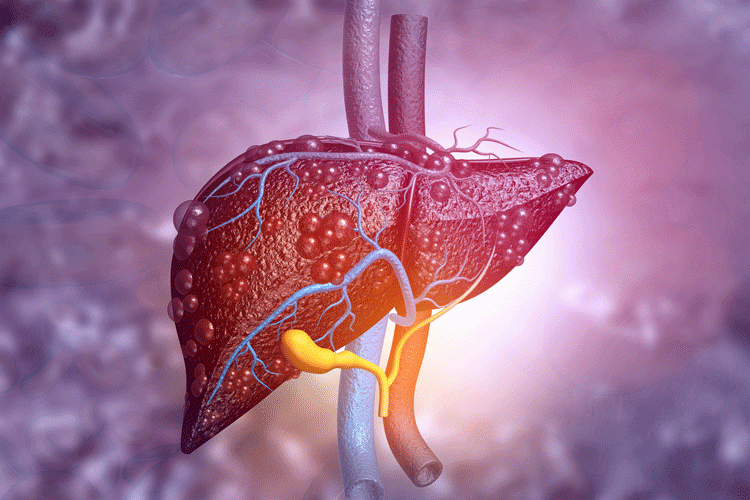
Non-alcoholic fatty liver disease (NAFLD), a growing epidemic leading to accumulation of triglycerides (TGs) and free fatty acids (FFAs) in the liver, comprises the early NAFL to its progressive form of non-alcoholic steatohepatitis (NASH).1,2 NAFLD prevalence, which has doubled over the last 20 years, now stands at 20-30 percent in Western countries; and the overall global prevalence is 20-25 percent, making NAFLD the leading cause of chronic liver diease.3-6 Currently, there are no US Food and Drug Administration (FDA)-approved drugs for NAFLD on the market.6 This is mostly due to the enormously complicated nature of NAFLD, highlighted by its recent label as a “multiple hit and organ” disease. The progression of the condition, its complexity and spatial and temporal heterogeneity need to be well understood in order to develop effective therapies.
NAFLD presents a progressive and heterogenous spectrum. It starts with isolated hepatocytes accumulating excessive amounts of lipids, involves other cell types throughout disease progression and can progress to more severe forms like NASH, fibrosis, cirrhosis and cancer (Figure 1). This initial accumulation is in response to the uptake of FFAs, dietary fats and de novo lipogenesis.7-9 Further progress to severe conditions is linked to inflammation (both locally and via interaction with gut and adipose tissues), insulin resistance and alterations to lipid homeostasis. These eventually induce a cascade through oxidative and endoplasmic reticulum (ER) stress in hepatocytes (lipotoxicity) and release of damage-associated molecular patterns that activate kupffer cells (KCs) and hepatic stellate cells (HSCs), additionally promoting inflammation and fibrosis.6,10,11
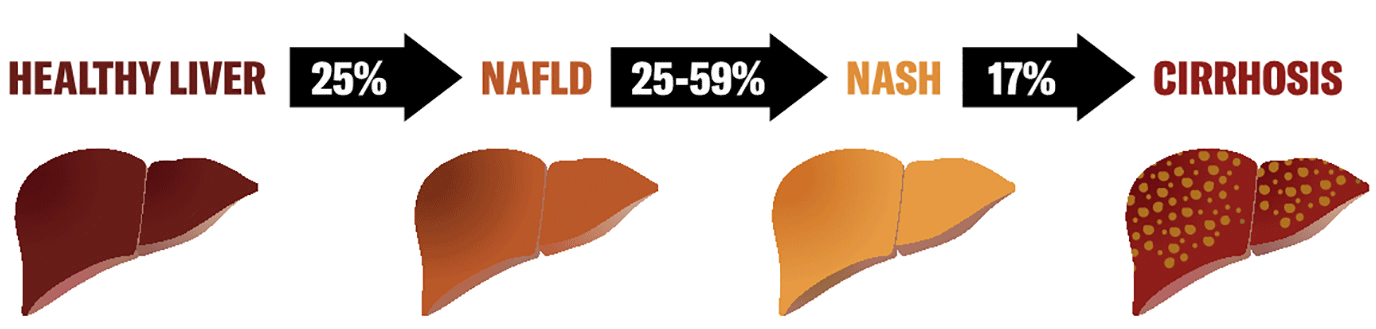
Figure 1: Incidence of NAFLD progression: from healthy liver to cirrhosis. Figure designed by and courtesy of Carolina Rey-Bedon, Milan, Italy.
Activated KCs produce inflammatory cytokines (TNF-a, IL-1b and IL-6, among others) that further injure hepatocytes and factors (TGF-a, PDGF) that stimulate HSCs.12,13 Recent work also indicates that liver sinusoidal endothelial cell (LSEC) injury appears during the simple steatosis phase and might have a “gate-keeper” role in the progression from simple steatosis to NASH and that their injury may be necessary for KC and HSC activation, resulting in the vicious injury cascade.14
NAFLD, especially as macrosteatotis prevails and the organ progresses towards NASH, presents high complexity involving not one but many independent and interconnected pathways via not only the hepatocytes but also the non‑parenchymal cells. In the previous decade, most emerging NAFLD/NASH drugs targeted one specific symptom via one pathway; thus, their successes have been limited. Armed with our updated collective understanding of the more complex nature of the disease spectrum, a new frontier in NAFLD/NASH treatment has emerged, using a combination of therapies with two or more drugs targeting different pathways. This approach has recently gained considerable momentum and over 20 such combinatorial clinical trials are now underway (https:// clinicaltrials.gov/). A few examples of such trials include Pioglitazone-empagliflozin (NCT03646292), Rosiglitazone-Metformin (NCT00699036), Semaglutide-Firsocostat-Cilofexor (NCT03987074), with others like Obeticholic Acid-Elafibranor currently in animal studies.15 Thus, efficient dose testing of such combinations is an urgent and growing need. Another challenging aspect of this complex disease is its heterogeneity manifesting itself as time-dependent or spatial heterogeneity of the symptoms, which was captured by a recent mass spectrometry imaging study (MALDI-MSI), demonstrating distinct lipid compositions at various disease stages.16 A more recent study shows the zonal differences in the length of TGs stored and the enrichment of macrosteatotic hepatocytes in saturated TGs compared to microsteatotic ones.17
In order to understand the pathogenesis of this complicated disease and to test existing and emerging drug candidates, several in vitro NAFLD models have been developed over the past decades. Some of the shortcomings of these in vitro models have traditionally been the lack of perfusion to imitate in vivo blood flow, use of high glucose and insulin concentrations not representing in vivo conditions, use of cell lines instead of primary cells, focusing on a single cell type – usually the parenchymal cells of the liver – and most importantly, concentrating only on a single phase of the disease instead of capturing the spatial heterogeneity.18-24 There have, however, been several in vitro studies that have propelled us in the right direction. For example, Feaver et al.7 and Davidson et al.8 have both used multiple cell types for recapitulating the cellular complexity of NAFLD and used more physiological glucose levels for their basal media to better mimic the in vivo microenvironment. Furthermore, Feaver et al. used a perfused macroscopic culture environment (the Hemoshear platform) for up to 14 days, where they intended to recapitulate NAFL and NASH signatures. An exciting approach in this study was the one-to-one omics correlation approach between existing patient data and the in vitro platform itself. Nevertheless, the throughput of the method used in this study was quite low, costly and utilised proprietary machinery. An important progress in terms of addressing the multi-organ theory, the interaction between different tissues involved in NAFLD, was demonstrated by Lee et al. where they studied the gut-liver axis in a two-compartment microfluidic chip to understand the absorption of free acids by the gut and their subsequent metabolism by the liver. A downside of this work was the use of cell lines in place of primary gut and liver parenchymal cells.
Despite this progress, current platforms, most of which are in their infancy, neither provide the high fidelity (different aspects of NAFLD and its spatial and temporal heterogeneity) nor the throughput to efficiently perform dose-response studies for combinatorial therapy approaches. In vitro recapitulation of such heterogeneity in NAFLD is currently lacking, which is of great importance in order to understand the disease mechanism and progression; and to advance target leads in the development of effective treatment approaches. In our recent work (Figure 2), we established an in vitro progressive NAFLD on a chip platform using a free fatty acid gradient to capture a spectrum of disease conditions in a continuous tissue.25 Through this microfluidic platform, we were able to show the possibility of increasingly severe lipid accumulation by metabolic patterning and also investigate the effect of oxygen gradients in NAFLD resulting in zonal lipid accumulation25 – similar to what is observed in vivo.26 While not demonstrated yet, and restriction of the platform being limited to five channels, ie, conditions, it can be used to test candidate drugs either at a single concentration or in a linearly increasing fashion in response to the disease severity. Combinations of drugs can also be analysed either in proportionally increasing or inversely decreasing concentrations by creatively modulating the extreme ends of the gradient generator inputs. Furthermore, increase of the throughput in such a model can be achieved by raising the channel number and multiplexing cross gradient chambers for testing even more conditions. An important advantage of such platforms via gradient microfluidic approaches is the reduced number of pumps per condition tested towards reducing the footprint of the in vitro platform.
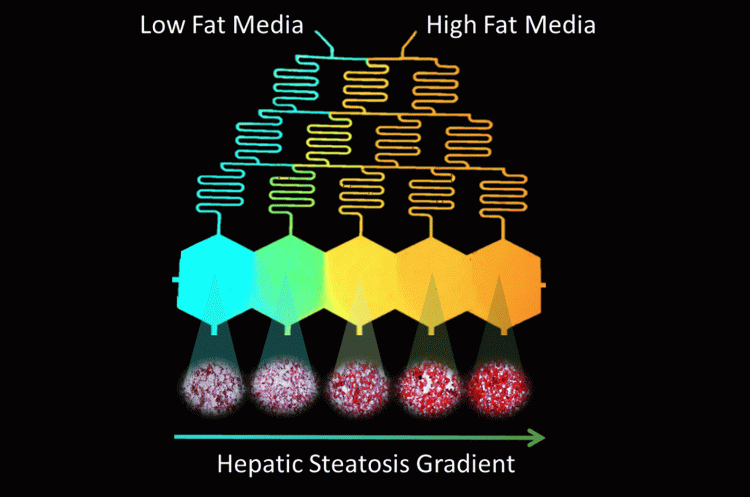
Figure 2: Design of Microfluidic-Pattern-on-a-Chip (MPOC) device creating a gradient from low fat (blue) to high fat (orange) media across hexagonal cell culture chambers. This creates a progressive accumulation of lipids in the cultured hepatocytes, visualised via Oil-Red-O Staining.
The throughput in NAFLD/NASH models is especially important given the two discussed complications of this disease. These are a) the emerging new paradigm of combinatorial therapies, which greatly increases the number of conditions requiring testing and b) the highly heterogenous nature of the disease in a single tissue. Microfluidic in vitro tissue culture platforms are already advantageous due to their physiologically relevant dimensions and perfusability. We posit that they are also ideal for establishing practically useful NAFLD/NASH models since they can also be engineered for high throughput where a combination of multiple drugs and disease stages can be tested simultaneously.
In addition to our own vision of extending our NAFLD platform to 64 or more simultaneous conditions using only four input pumps and with multiple cell types, there are many exciting new studies in this arena. A promising platform is from the Draper Laboratories, where their 96 well individually addressable platform can maintain hepatic cultures stably for 14 days.27 We believe that the next decade will increase the relevance, fidelity (spatial and temporal heterogeneity) and throughput of in vitro platforms for NAFLD drug efficacy testing via the use of microfluidics. This, in turn, is expected to aid and accelerate the quest for identifying better treatments for this debilitating disease in a more efficient and cost‑effective manner.
About the authors
Beyza Bulutoglu has a PhD in Chemical Engineering from Columbia University and an MS in Biomedical Engineering from Yale University. She completed her postdoctoral studies at the Center of Engineering in Medicine at Massachusetts General Hospital, where she spearheaded multiple projects, including the establishment of disease-on-a-chip platforms to study NAFLD progression. Beyza has a strong background in genetic and protein engineering and contributed to projects in a broad range of research areas including synthetic biology and tissue engineering. She recently joined Genentech, where her group develops large molecule therapeutics for effective therapies in various areas including cancer immunology.
Camilo Rey-Bedón is a technician at the Massachusetts General Hospital. At the Center for Engineering in Medicine, his research focuses on studying the effect of fatty liver disease on drug metabolism, the development of organ-on-a-chip disease models and the deep supercooling of liver organoids. His research interests lie in the application of novel microfabrication and molecular technologies to mimic the complexity of human biology in vitro.
Peony Banik’s research at the Center for Engineering in Medicine explores the effect of fatty liver diseases on drug metabolism. She is also involved in projects focusing on prolonging the time transplantable human livers remain alive ex vivo via cryopreservation. Her interests lie in creating and developing biotechnologies in the field of toxicology and transplant surgery.
Aslihan Gokaltun received a bachelor’s degree in chemical engineering from Hacettepe University in Turkey. She went on to gain her MS and PhD degrees in chemical engineering through the programme in Polymer Science and Technology at Hacettepe University. Currently, she is working as a postdoctoral fellow in the Center for Engineering in Medicine and Surgery at Harvard Medical School. Her research interests focus on the design and synthesis of new materials for organs-on-chips and tissue engineering applications.
Martin L Yarmush is the founding director of the Center for Engineering in Medicine and the Massachusetts General Hospital and the Harvard Medical School. He is a world-renowned expert in the broad fields of biomedical engineering, tissue engineering and biopreservation. Martin’s investigative activities encompass both basic and applied research and have resulted in numerous patents and the formation of over 10 start-up companies.
Osman Berk Usta is an Assistant Professor of Surgery and Bioengineering at the Harvard Medical School and Massachusetts General Hospital as part of the Center for Engineering in Medicine. His research interests lie at the intersection of (micro)-engineered tissue models for healthy and diseased physiologies, biopreservation and computational modelling. He and his team have been developing complex liver tissue models to mimic different aspects of liver physiology such as zonation and they have more recently developed models mimicking progression of non-alcoholic fatty liver disease.
References
1. Angulo, P.; Lindor, K. D., Non-alcoholic fatty liver disease. J Gastroenterol Hepatol 2002, 17, S186-S190.
2. Benedict, M.; Zhang, X., Non-alcoholic fatty liver disease: An expanded review. World J Hepatol 2017, 9 (16), 715-732.
3. Younossi, Z.; Anstee, Q. M.; Marietti, M.; Hardy, T.; Henry, L.; Eslam, M.; George, J.; Bugianesi, E., Global burden of NAFLD and NASH: trends, predictions, risk factors and prevention. Nat Rev Gastroenterol Hepatol 2018, 15 (1), 11-20.
4. LaBrecque, D. R.; Abbas, Z.; Anania, F.; Ferenci, P.; Khan, A. G.; Goh, K. L.; Hamid, S. S.; Isakov, V.; Lizarzabal, M.; Penaranda, M. M.; Ramos, J. F.; Sarin, S.; Stimac, D.; Thomson, A. B.; Umar, M.; Krabshuis, J.; LeMair, A., World Gastroenterology Organisation global guidelines: Nonalcoholic fatty liver disease and nonalcoholic steatohepatitis. J Clin Gastroenterol 2014, 48 (6), 467-73.
5. Rinella, M.; Charlton, M., The globalization of nonalcoholic fatty liver disease: Prevalence and impact on world health. Hepatology 2016, 64 (1), 19-22.
6. Diehl, A. M.; Day, C., Cause, Pathogenesis, and Treatment of Nonalcoholic Steatohepatitis. N Engl J Med 2017, 377 (21), 2063-2072.
7. Feaver, R. E.; Cole, B. K.; Lawson, M. J.; Hoang, S. A.; Marukian, S.; Blackman, B. R.; Figler, R. A.; Sanyal, A. J.; Wamhoff, B. R.; Dash, A., Development of an in vitro human liver system for interrogating nonalcoholic steatohepatitis. JCI Insight 2016, 1 (20), e90954.
8. Sanders, F. W.; Griffin, J. L., De novo lipogenesis in the liver in health and disease: more than just a shunting yard for glucose. Biol Rev Camb Philos Soc 2016, 91 (2), 452-68.
9. Hellerstein, M. K., De novo lipogenesis in humans: metabolic and regulatory aspects. Eur J Clin Nutr 1999, 53 Suppl 1, S53-65.
10. Fierbinteanu-Braticevici, C.; Sinescu, C.; Moldoveanu, A.; Petrisor, A.; Diaconu, S.; Cretoiu, D.; Braticevici, B., Nonalcoholic fatty liver disease: one entity, multiple impacts on liver health. Cell Biol Toxicol 2017, 33 (1), 5-14.
11. Di Maso, V.; Bellentani, S., Is there an effective therapy available for non-alcoholic fatty liver disease? F1000 Med Rep 2009, 1.
12. Heymann, F.; Tacke, F., Immunology in the liver–from homeostasis to disease. Nat Rev Gastroenterol Hepatol 2016, 13 (2), 88-110.
13. Arrese, M.; Cabrera, D.; Kalergis, A. M.; Feldstein, A. E., Innate Immunity and Inflammation in NAFLD/ NASH. Dig Dis Sci 2016, 61 (5), 1294-303.
14. Miyao, M.; Kotani, H.; Ishida, T.; Kawai, C.; Manabe, S.; Abiru, H.; Tamaki, K., Pivotal role of liver sinusoidal endothelial cells in NAFLD/NASH progression. Lab Invest 2015, 95 (10), 1130-44.
15. Roth, J. D.; Veidal, S. S.; Fensholdt, L. K. D.; Rigbolt, K. T. G.; Papazyan, R.; Nielsen, J. C.; Feigh, M.; Vrang, N.; Young, M.; Jelsing, J.; Adorini, L.; Hansen, H. H., Combined obeticholic acid and elafibranor treatment promotes additive liver histological improvements in a diet-induced ob/ob mouse model of biopsy-confirmed NASH. Sci Rep 2019, 9 (1), 9046.
16. Scupakova, K.; Soons, Z.; Ertaylan, G.; Pierzchalski, K. A.; Eijkel, G. B.; Ellis, S. R.; Greve, J. W.; Driessen, A.; Verheij, J.; De Kok, T. M.; Olde Damink, S. W. M.; Rensen, S. S.; Heeren, R. M. A., Spatial Systems Lipidomics Reveals Nonalcoholic Fatty Liver Disease Heterogeneity. Anal Chem 2018, 90 (8), 5130-5138.
17. Alamri, H.; Patterson, N. H.; Yang, E.; Zoroquiain, P.; Lazaris, A.; Chaurand, P.; Metrakos, P., Mapping the triglyceride distribution in NAFLD human liver by MALDI imaging mass spectrometry reveals molecular differences in micro and macro steatosis. Anal Bioanal Chem 2019, 411 (4), 885-894.
18. Davidson, M. D.; Ballinger, K. R.; Khetani, S. R., Long-term exposure to abnormal glucose levels alters drug metabolism pathways and insulin sensitivity in primary human hepatocytes. Sci Rep 2016, 6, 28178.
19. Lee, S. Y.; Sung, J. H., Gut-liver on a chip toward an in vitro model of hepatic steatosis. Biotechnol Bioeng 2018.
20. Mashek, D. G.; Grummer, R. R., Effects of long chain fatty acids on lipid and glucose metabolism in monolayer cultures of bovine hepatocytes. J Dairy Sci 2003, 86, 2390-2396.
21. Ricchi, M.; Odoardi, M. R.; Carulli, L.; Anzivino, C.; Ballestri, S.; Pinetti, A.; Fantoni, L. I.; Marra, F.; Bertolotti, M.; Banni, S.; Lonardo, A.; Carulli, N.; Loria, P., Differential effect of oleic and palmitic acid on lipid accumulation and apoptosis in cultured hepatocytes. J Gastroenterol Hepatol 2009, 24 (5), 830-40.
22. Janorkar, A. V.; King, K. R.; Megeed, Z.; Yarmush, M. L., Development of an in vitro cell culture model of hepatic steatosis using hepatocyte-derived reporter cells. Biotechnol Bioeng 2009, 102 (5), 1466-74.
23. Zhao, F.; Xie, P.; Jiang, J.; Zhang, L.; An, W.; Zhan, Y., The effect and mechanism of tamoxifen-induced hepatocyte steatosis in vitro. Int J Mol Sci 2014, 15 (3), 4019-30.
24. Gori, M.; Simonelli, M. C.; Giannitelli, S. M.; Businaro, L.; Trombetta, M.; Rainer, A., Investigating Nonalcoholic Fatty Liver Disease in a Liver-on-a-Chip Microfluidic Device. PLoS One 2016, 11 (7), e0159729.
25. Bulutoglu, B.; Rey-Bedon, C.; Kang, Y. B. A.; Mert, S.; Yarmush, M. L.; Usta, O. B., A microfluidic patterned model of non-alcoholic fatty liver disease: applications to disease progression and zonation. Lab Chip 2019, 19 (18), 3022-3031.
26. Hall, Z.; Bond, N. J.; Ashmore, T.; Sanders, F.; Ament, Z.; Wang, X.; Murray, A. J.; Bellafante, E.; Virtue, S.; Vidal-Puig, A.; Allison, M.; Davies, S. E.; Koulman, A.; Vacca, M.; Griffin, J. L., Lipid zonation and phospholipid remodeling in nonalcoholic fatty liver disease. Hepatology 2017, 65 (4), 1165-1180.
27. Tan, K.; Keegan, P.; Rogers, M.; Lu, M.; Gosset, J. R.; Charest, J.; Bale, S. S., A high-throughput microfluidic microphysiological system (PREDICT-96) to recapitulate hepatocyte function in dynamic, re-circulating flow conditions. Lab Chip 2019, 19 (9), 1556-1566.
Related topics
Analysis, Analytical Techniques, Disease Research, Drug Discovery, Drug Targets, High-Throughput Screening (HTS), In Vitro, Microfluidic Technology, Organ-on-a-Chip, Organoids, Research & Development, Screening, Therapeutics
Related conditions
cirrhosis, Liver cancer, Liver fibrosis, non-alcoholic fatty liver disease (NAFLD), Non-alcoholic steatohepatitis (NASH)
Related organisations
ForteBio, Horizon Discovery, Merck, Ncardia, US Food and Drug Administration (FDA)