Nuclear Magnetic Resonance as a tool for chemokine inhibitor discovery
Posted: 1 May 2014 | Brian Volkman (Department of Biochemistry: Medical College of Wisconsin)
Chemokines orchestrate leukocyte trafficking in the immune system, but unregulated or inappropriate chemokine activity plays a key role in many inflammatory, allergic and autoimmune diseases as well as metastatic tumour progression. The chemokine network is comprised of ~50 distinct ligands that specifically bind and activate a family of 20 G protein-coupled receptors (GPCRs), many of which are targets for drugs at various stages of development…
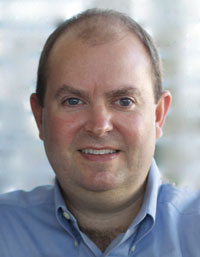
Brian F. Volkman, Department of Biochemistry, Medical College of Wisconsin
Two FDA-approved chemokine receptor antagonists are currently in clinical use: Maraviroc (Pfizer); a CCR5 antagonist for HIV infection, and Plerixafor (Genzyme/Sanofi-Aventis); a CXCR4 antagonist for stem cell mobilisation. Drug candidates targeting at least half of the known chemokine receptors are currently in human trials for many other clinical indications, including rheumatoid arthritis, atherosclerosis, multiple sclerosis, diabetic nephropathy, asthma, and multiple cancer types. Like Maraviroc and Plerixafor, most of these drugs are small molecule GPCR antagonists that emerged from high-throughput screening (HTS) efforts. However, biologic drugs are also in development, including peptides, monoclonal antibodies, antisense oligonucleotides and zinc finger nucleases.
Other chemokines continue to be newly validated as targets for diseases that impose a significant healthcare burden. For example, psoriasis is a common inflammatory skin condition that affects two to five per cent of the world’s population with an economic cost of treatment approaching several billion US dollars3. Likewise, asthma affects over eight per cent of the population4 and has increased in the US and other modernised countries5,6. Recent work by Hwang and colleagues found that the chemokine CCL20 and its receptor CCR6 are key drivers of the epidermal inflammation and hyperplasia observed in psoriasis, and that antibody blockade of CCL20 can prevent the development of disease7. Respiratory viral infections have been implicated in both the development of asthma and its exacerbation8, and lung-specific production of the chemokine CCL28 is a critical inflammatory mediator. Grayson and colleagues showed that an anti-CCL28 monoclonal antibody prevents post-viral asthma, validating this chemokine as a target for the development of new therapeutics9.
GPCR-directed drugs typically bind the orthosteric site, a deep pocket in the heptahelical transmembrane (7TM) domain, and prevent binding and activation by the endogenous ligand. In contrast to typical small molecule or peptide GPCR agonists, chemokines engage their receptors using an extensive protein-protein interface with two distinct parts10. Chemokines are small (~10 kDa) secreted proteins that adopt a unique conformation – the chemokine fold – which binds an epitope in the flexible N-terminal domain of the GPCR (site 1). The chemokine N-terminus then docks into the orthosteric 7TM pocket (site 2) analogous to other GPCR agonists.
Notwithstanding this well-established two-step / two-site model, most drug discovery against the chemokine network has used high-throughput screening without the benefit of structural details of the chemokine-GPCR protein-protein interface to identify antagonists that bind site 2.
While an intact chemokine-receptor complex has yet to be solved, recent NMR11 and x-ray crystal structures12,13 have separately revealed important details of the two interaction sites that can be exploited for structure-based drug discovery (SBDD). Among integral membrane proteins, the GPCRs have been especially challenging targets for SBDD, but the prospects are improving with recent advances in structural methods. Determination of the b2-adrenergic receptor (b2-AR) crystal structure14 was immediately followed by structure-based discovery of new inhibitors of this well-known GPCR drug target15. Similar in silico screening for ligands that bind the orthosteric pocket (site 2) of the first chemokine receptor structure12 yielded CXCR4 inhibitors with novel chemotypes and micromolar potency16.
Ideally, intact chemokine receptors would also be subjected to NMR-based experimental screening for new ligands, but integral membrane proteins are often intractable due to their limited solubility and loss of native structure and function upon detergent extraction and purification. Relatively few NMR studies of intact GPCRs have been published. Opella and colleagues solved the structure of the chemokine receptor CXCR1 in liquid crystalline phospholipid bilayers by NMR17, and Kobilka and colleagues used NMR of selectively 13C-labeled b2-AR to monitor ligand binding and detect functionally important changes in GPCR conformation and dynamics in solution18,19. While NMR-based screening for new chemokine receptor ligands may be on the horizon, GPCRs remain daunting targets. However, the chemokine ligands might be ideal targets for NMR-based screening and drug discovery methods if a site for inhibition can be identified and shown to be ‘druggable’. The NMR structure of a CXCR4 N-terminal fragment bound to CXCL1211 provided the first suggestion that specific site 1 interactions could be targeted on the chemokine itself.
Chemokine-receptor contacts at site 1 typically involve a sulfotyrosine modification in the N-terminal domain of the receptor20. Veldkamp and coworkers used NMR to solve the structure of a CXCR4 N-terminal sulfopeptide bound to CXCL12 and identified three potential sulfotyrosine recognition sites on the CXCL12 surface11. Subsequent work showed that one of the three CXCR4 sulfotyrosine modifications (at residue 21) contributed the most to the site 1 binding affinity and specificity21. A structural bioinformatic analysis suggested that this sulfotyrosine binding site is conserved across the chemokine family22. This led to the hypothesis that a small molecule ligand bound to this site on the chemokine surface could disrupt receptor binding and inhibit chemokine signalling.
NMR spectroscopy is sensitive to changes in molecular structure at atomic resolution. Consequently, it has been widely used to detect the interactions of macromolecules with small molecule ligands and other binding partners. NMR is well established as a screening tool for drug discovery that can monitor either the target or ligand for evidence of binding23. The fragment-based screening approach pioneered by Fesik and coworkers (‘SAR by NMR’)24 uses NMR to monitor ligand-induced changes in the 1H, 15N and/or 13C chemical shifts in a protein target of known structure, and variations on this approach are widely used in academic and industrial SBDD25. Chemokines like CXCL12 typically have well-resolved 2D NMR spectra, which enables sensitive detection of ligand binding26. Each amino acid in the protein has a unique spectral identifier or ‘crosspeak’ arising at the intersection of its amide 1H and 15N chemical shifts. Addition of a small molecule ligand will shift a subset of the crosspeaks to new positions in the 2D NMR spectrum. The magnitude of these changes or ‘chemical shift perturbations’ can be used to highlight the regions of the protein which are most affected by the binding reaction, a procedure called chemical shift mapping.
If the desired binding site for drug discovery is known, computational docking to experimentally determined protein structures can be used to screen large ‘virtual’ compound databases to prioritise molecules for functional tests and improve hit rates more than 200-fold. Veldkamp and co-workers used chemical shift mapping to identify a specific sulfotyrosine binding pocket on the CXCL12 surface27 and used this structural information as input for high-throughput docking calculations. Virtual screening yielded a list of candidate ligands from the ZINC library, a free database of commercially available compounds created for the purpose of computational screening28. They purchased a subset of the top-ranked molecules for experimental screening by 2D NMR to identify ligands that bind specifically to the sulfotyrosine recognition site.
Veldkamp et al. measured 2D NMR spectra of CXCL12 in the presence of each compound selected from the docking results, and found that a most of them bound specifically to the conserved sulfotyrosine binding cleft. The initial hits were fragment-like molecules with low (millimolar) affinity, except for one larger lead-like molecule that bound more tightly and induced the largest chemical shift perturbations in the CXCL12 NMR spectrum. The hit molecule with the highest affinity for CXCL12 was a 350 Da thiourea-linked napthyl- and benzoic acid-containing compound (ZINC ID 310454). Subsequent testing in cell-based assays for chemokine function showed that this compound could also inhibit CXCL12 activation of its receptor CXCR4 with no effect on other chemokine-induced signalling. A follow-up study by the same group showed that inhibitors with improved ligand efficiency could be synthesised by substituting different linkers and functional groups that preserved key polar and apolar contacts with the chemokine29. Another small molecule CXCL12 inhibitor, the neutralising ligand (‘neutraligand’) chalcone 4, has been reported, but the structural basis for its activity has not been demonstrated30. Thus, inhibition of CXCR4-mediated in vitro cell migration by small molecule ligands for CXCL12 represents the first proof of principle for structure-based discovery of inhibitors that function by binding and neutralising the chemokine instead of its cognate GPCR.
Chemokines are unconventional targets for drug discovery, given their small size and absence of the deep clefts or pockets typical of ligand or substrate binding sites in enzymes and receptors. Additional optimisation will be required to generate inhibitors of sufficient potency to be effective in vivo. In this respect, chemokines are like other protein-protein interface drug targets, where fragment-linking strategies have yielded potent inhibitors of Bcl-2 family proteins31 and the cytokine interleukin-232.
Despite the obvious challenges, NMR-guided discovery and development of small molecule inhibitors of CXCL12 and other chemokines will benefit from a few unique advantages. First, sulfotyrosine is a conserved element of the site 1 chemokine-receptor interface, and NMR chemical shift mapping can pinpoint the druggable sites on the chemokine surface using sulfopeptide probes derived from the GPRC N-terminal domain27. Second, the successful discovery of a CXCL12 inhibitor by virtual screening demonstrated that NMR structures of soluble chemokine-peptide ‘site 1’ complexes are suitable templates for high-throughput docking of virtual compound libraries. Additionally, automated NMR screening using robots for sample preparation and automated data acquisition can further accelerate the validation of chemokine ligands. Finally, NMR detection of ligand binding at structurally distinct sub-sites can facilitate the use of fragment linking to build inhibitor potency and selectivity. In summary, a growing number of chemokines and their receptors are important therapeutic targets, and NMR is a powerful tool for structure-based ligand discovery that will contribute to many stages of the drug discovery process.
References
- Garin A, Proudfoot AE. Chemokines as targets for therapy. Exp Cell Res. 2011;317(5):602-12
- Bachelerie F, Ben-Baruch A, Burkhardt AM, Combadiere C, Farber JM, Graham GJ, et al. International Union of Pharmacology. LXXXIX. Update on the extended family of chemokine receptors and introducing a new nomenclature for atypical chemokine receptors. Pharmacol Rev. 2014;66(1):1-79
- Hedrick MN, Lonsdorf AS, Hwang ST, Farber JM. CCR6 as a possible therapeutic target in psoriasis. Expert Opin Ther Targets. 2010;14(9):911-22
- Sly RM. Changing prevalence of allergic rhinitis and asthma. Annals of allergy, asthma & immunology : official publication of the American College of Allergy, Asthma, & Immunology. 1999;82(3):233-48; quiz 48-52
- Vital signs: asthma prevalence, disease characteristics, and self-management education: United States, 2001–2009. MMWR Morbidity and mortality weekly report. 2011;60(17):547-52
- Asher MI, Montefort S, Bjorksten B, Lai CK, Strachan DP, Weiland SK, et al. Worldwide time trends in the prevalence of symptoms of asthma, allergic rhinoconjunctivitis, and eczema in childhood: ISAAC Phases One and Three repeat multicountry cross-sectional surveys. Lancet. 2006;368(9537):733-43
- Hedrick MN, Lonsdorf AS, Shirakawa AK, Richard Lee CC, Liao F, Singh SP, et al. CCR6 is required for IL-23-induced psoriasis-like inflammation in mice. J Clin Invest. 2009;119(8):2317-29
- Kumar A, Grayson MH. The role of viruses in the development and exacerbation of atopic disease. Annals of allergy, asthma & immunology : official publication of the American College of Allergy, Asthma, & Immunology. 2009;103(3):181-6; quiz 6-7, 219
- Grayson MH, Cheung D, Rohlfing MM, Kitchens R, Spiegel DE, Tucker J, et al. Induction of high-affinity IgE receptor on lung dendritic cells during viral infection leads to mucous cell metaplasia. J Exp Med. 2007;204(11):2759-69. PMCID: 2118483
- Crump MP, Gong JH, Loetscher P, Rajarathnam K, Amara A, Arenzana-Seisdedos F, et al. Solution structure and basis for functional activity of stromal cell-derived factor-1; dissociation of CXCR4 activation from binding and inhibition of HIV-1. EMBO J. 1997;16(23):6996-7007. PMCID: 1170303
- Veldkamp CT, Seibert C, Peterson FC, De la Cruz NB, Haugner JC, 3rd, Basnet H, et al. Structural basis of CXCR4 sulfotyrosine recognition by the chemokine SDF-1/CXCL12. Science signaling. 2008;1(37):ra4
- Wu B, Chien EY, Mol CD, Fenalti G, Liu W, Katritch V, et al. Structures of the CXCR4 chemokine GPCR with small-molecule and cyclic peptide antagonists. Science. 2010;330(6007):1066-71
- Tan Q, Zhu Y, Li J, Chen Z, Han GW, Kufareva I, et al. Structure of the CCR5 chemokine receptor-HIV entry inhibitor maraviroc complex. Science. 2013;341(6152):1387-90
- Cherezov V, Rosenbaum DM, Hanson MA, Rasmussen SG, Thian FS, Kobilka TS, et al. High-resolution crystal structure of an engineered human beta2-adrenergic G protein-coupled receptor. Science. 2007;318(5854):1258-65
- Kolb P, Rosenbaum DM, Irwin JJ, Fung JJ, Kobilka BK, Shoichet BK. Structure-based discovery of beta2-adrenergic receptor ligands. Proc Natl Acad Sci U S A. 2009;106(16):6843-8
- Mysinger MM, Weiss DR, Ziarek JJ, Gravel S, Doak AK, Karpiak J, et al. Structure-based ligand discovery for the protein-protein interface of chemokine receptor CXCR4. Proc Natl Acad Sci U S A. 2012;109(14):5517-22. PMCID: 3325704
- Park SH, Das BB, Casagrande F, Tian Y, Nothnagel HJ, Chu M, et al. Structure of the chemokine receptor CXCR1 in phospholipid bilayers. Nature. 2012;491(7426):779-83
- Bokoch MP, Zou Y, Rasmussen SG, Liu CW, Nygaard R, Rosenbaum DM, et al. Ligand-specific regulation of the extracellular surface of a G-protein-coupled receptor. Nature. 2010;463(7277):108-12
- Nygaard R, Zou Y, Dror RO, Mildorf TJ, Arlow DH, Manglik A, et al. The dynamic process of beta(2)-adrenergic receptor activation. Cell. 2013;152(3):532-42
- Choe H, Farzan M. Chapter 7. Tyrosine sulfation of HIV-1 coreceptors and other chemokine receptors. Methods Enzymol. 2009;461:147-70
- Ziarek JJ, Getschman AE, Butler SJ, Taleski D, Stephens B, Kufareva I, et al. Sulfopeptide Probes of the CXCR4/CXCL12 Interface Reveal Oligomer-Specific Contacts and Chemokine Allostery. ACS Chem Biol. 2013;8(9):1955-63. PMCID: 3783652
- Ziarek JJ, Heroux MS, Veldkamp CT, Peterson FC, Volkman BF. Sulfotyrosine recognition as marker for druggable sites in the extracellular space. Int J Mol Sci. 2011;12(6):3740-56
- Harner MJ, Frank AO, Fesik SW. Fragment-based drug discovery using NMR spectroscopy. J Biomol NMR. 2013;56(2):65-75
- Hajduk PJ, Meadows RP, Fesik SW. Discovering high-affinity ligands for proteins. Science. 1997;278(5337):497,9
- Campos-Olivas R. NMR screening and hit validation in fragment based drug discovery. Curr Top Med Chem. 2011;11(1):43-67
- Ziarek JJ, Volkman BF. NMR in the Analysis of Functional Chemokine Interactions and Drug Discovery. Drug discovery today Technologies. 2012;9(4):e293-e9. PMCID: 3499625
- Veldkamp CT, Seibert C, Peterson FC, Sakmar TP, Volkman BF. Recognition of a CXCR4 sulfotyrosine by the chemokine stromal cell-derived factor-1alpha (SDF-1alpha/CXCL12). J Mol Biol. 2006;359(5):1400-9
- Veldkamp CT, Ziarek JJ, Peterson FC, Chen Y, Volkman BF. Targeting SDF-1/CXCL12 with a Ligand That Prevents Activation of CXCR4 through Structure-Based Drug Design. J Am Chem Soc. 2010
- Ziarek JJ, Liu Y, Smith E, Zhang G, Peterson FC, Chen J, et al. Fragment-based optimization of small molecule CXCL12 inhibitors for antagonizing the CXCL12/CXCR4 interaction. Curr Top Med Chem. 2012;12(24):2727-40
- Hachet-Haas M, Balabanian K, Rohmer F, Pons F, Franchet C, Lecat S, et al. Small neutralizing molecules to inhibit actions of the chemokine CXCL12. J Biol Chem. 2008;283(34):23189-99
- Hajduk PJ. SAR by NMR: putting the pieces together. Mol Interv. 2006;6(5):266-72.
- Arkin MR, Randal M, DeLano WL, Hyde J, Luong TN, Oslob JD, et al. Binding of small molecules to an adaptive protein-protein interface. Proc Natl Acad Sci U S A. 2003;100(4):1603-8
Biography
Brian Volkman is Professor of Biochemistry at the Medical College of Wisconsin. He trained as a protein NMR spectroscopist at the University of New South Wales, UC Berkeley and UW–Madison. Since 2000, he has led an NIH-funded structural biology research programme on chemokine signalling and inhibition. His group solved the NMR structure of the first complex between a chemokine and a sulfotyrosine-modified receptor fragment. An engineered chemokine dimer they originally created as a tool for structural studies is now a patented CXCR4 inhibitor that blocks tumour cell migration and prevented metastatic progression in animal models of colorectal carcinoma and metastatic melanoma. Based on the structural details of sulfotyrosine-recognition in the chemokine-receptor interface, Dr Volkman developed a novel small molecule inhibitor discovery platform. His group uses a hybrid in silico / NMR screening strategy to target this conserved recognition site to identify small molecules that bind the chemokine and block receptor binding. In addition to leading structure-based efforts to inhibit chemokines that promote metastatic, autoimmune or inflammatory pathologies, Dr Volkman recently co-founded Protein Foundry, LLC, a manufacturer of recombinant proteins for use in laboratory research and pre-clinical studies. [email protected]
Related topics
Drug Discovery, GPCRs, High-Throughput Screening (HTS), Nuclear Magnetic Resonance (NMR), Screening
Related conditions
HIV, Multiple Sclerosis
Related organisations
Medical College of Wisconsin
Related people
Brian Volkman