How microfluidics can automate drug discovery and development
Posted: 17 May 2021 | Dr Ruitao Su (University of Minnesota) | No comments yet
Researchers from the University of Minnesota have developed a 3D spherical microfluidic device. Here, Dr Ruitao Su explains how the new device can be used in drug discovery and development.
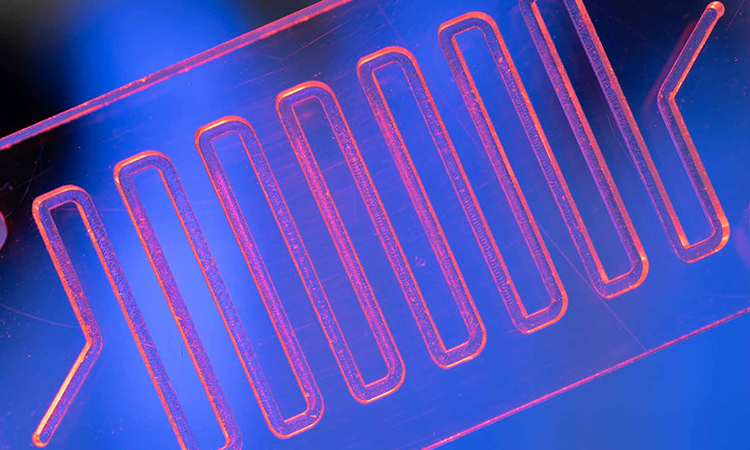
Researchers at the University of Minnesota, US, have 3D printed microfluidic channels on a curved surface. Led by Professor Michael McAlpine, the team say their study, published in Science Advances, provides an initial step for printing these channels directly on the skin for real-time sensing of bodily fluids. In this Q&A, Dr Ruitao Su explains how this microfluidic device can aid drug discovery and development.
How can advancements in the field of microfluidics aid drug discovery and development?
Drug screening conventionally requires rigorous animal tests, which are costly and time-consuming processes and also face ethic obstacles. The development of microfluidic technologies facilitates the precise control over drug concentrations and dose supply on the molecular and cellular levels. When used for the precise manipulation of fluidic chemical agents on the micro scale, microfluidic systems can detect the effect of drugs on cells and tissues under controlled conditions. Therefore, microfluidics provides a more efficient, economic and easy-to-implement method for drug discovery and development.
Can you describe the microfluidics platform you created? What can it be used for?
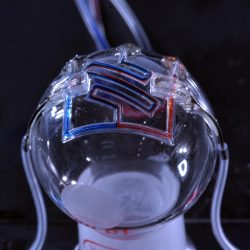
Researchers at the University of Minnesota are the first to 3D print microfluidic channels on a curved surface, providing the initial step for someday printing them directly on the skin for real-time sensing of bodily fluids [credit: McAlpine Group, University of Minnesota].
This project started with the goal of three-dimensionally (3D) printing multiplexed biosensing platforms that can detect and discriminate between various lipopolysaccharide-containing bacteria in a high-throughput and real-time manner. To this end, we needed to integrate an array of heterogeneous sensors that were functionalised with probing molecules with 3D printed microfluidic networks that can guide the flow of analytes through the sensors. What we realised was that the existing methods for 3D printing microfluidic devices, such as stereolithography and inkjet printing, cannot meet this goal without introducing contamination to the sensors. In other words, the residual resins or sacrificial materials present in these devices are harmful to the functionalised sensors and will damage its efficacy for biochemical sensing. Therefore, we designed and printed this new type of microfluidic device that forms hollow channels and chambers by extruding viscoelastic inks, which can be directly aligned and printed on microsensors and other surfaces. Even though this technology was developed for biochemical sensing, it can be readily transferred to other applications such as drug screening, point-of-care devices, tissue engineering and even personalised wearables for health monitoring.
How did you 3D print fluid channels at the micron scale?
What enables the printing of the self-supporting microfluidics is the yield-stress behaviour of the viscoelastic ink, silicone gel. This property renders the printing of standing thin walls by stacking extruded filaments in a certain angular range and avoids structural collapsing that occurs in other printing methods. We designed the printing toolpaths in a manner that closed the walls on the top to form channel and chamber structures. The resolution of the channels is controlled by specifying the distances between two opposite standing walls, which proved to be a robust and highly repeatable methodology.
Why did you choose to utilise curved surfaces?
Numerous application scenarios such as on-body health monitors require microfluidic devices that are printed on human skin or textiles, which are highly irregular in morphology. Fabricating microfluidic devices that are conformal to curved surfaces is challenging for conventional soft lithography and other 3D printing methods.
The development of microfluidic technologies facilitates the precise control over drug concentrations”
However, the self-supporting and extrusion-based nature of this methodology can fit various freeform surfaces. As we have demonstrated, microfluidic networks that are printed on spherical surfaces can realise functionalities such as chemical species mixing and automation of the flow control. This new capability brings technological advances by directly printing microfluidics-based biosensors on biological surfaces or textiles for mobile and on-site sensing applications.
What tests did you conduct on using microfluidics platform?
We conducted three categories of tests for this new type of microfluidic device:
- Intrinsic figures of merit of the silicone channels including incline angle, resolution and burst pressure. Our results showed that these properties meet the requirements of most microfluidic applications.
- Performances of automation components including microfluidic valves and pumps, which are effective in control and automate the microfluidic flows
- Functionality demonstrations including chemical species mixers, microfluidics-based salinity sensors and microfluidic networks on curved surfaces. The latter two demonstrations were most compelling because they were never realised before by other methodologies and hold great promises for future applications.
Did you face any challenges during your research and how did you overcome these?
Like most successful scientific innovations, a good idea or hypothesis is always the most critical factor. This research faced the challenge of how to realise seamless integration of microfluidics and electronic sensors from the beginning. I came up with the hypothesis that self-supporting structures might be printable if we control the stacking angle in a certain range, even though such structures have been shown to often fail previously. After putting efforts in experiments and going through some trial-and-error, I found a printable range for silicone-based microfluidics. Then I came up with the mathematical model to explain the result, laying the theoretical foundation for this new technology.
What advantages does this platform have over previous ones for drug development?
Several advantages have been noticed for this new type of devices, including:
Volumetric efficiency
Previous devices typically require a large slab of cured polymer to encase the microfluidic channels, resulting a bulky final product for the devices.
High-throughput and multiplexed testing
Microfluidic channels just provide a network where fluid flows are enabled. For drug screening and biochemical sensing, functional compartments where cells or functional elements are housed need to be created. Our method can functionalise the compartments with an array of probe molecules/cells and subsequently enclose the devices with 3D printed microfluidic structures, all conducted on one printing platform. This will create multiplexed devices that can screen multiple target drugs with one device.
Elastomeric properties
Stereolithography and inkjet printing produce rigid devices by curing polymer resins with UV light. Instead, we print silicone gels under room temperature and form highly elastic devices after cured in air. The devices demonstrated a fracture strain of 350 percent, which is highly beneficial to application that require a large deformation of the screening platforms.
Conformal to substrates
As the first demonstration of microfluidic networks on curved surfaces, this new type of microfluidic devices can be customised based on the geometry of the biological models and build true 3D structures.
Where do you see the future of microfluidics is headed?
Microfluidic devices are the main tool for us to test and conduct drug screening for the treatment of disease. Being able to customise and 3D print microfluidic structures directly on testing sensors will gave us a much more powerful tool for this purpose.
This technology is currently actively pursued for its applications in research labs, such as drug testing, organ-on-a-chip models and biomedical sensing. With the development of 3D printed conformal microfluidics, future microfluidic devices will be available to most people as wearable devices or “tattoos” that are printed on the skin for real-time health monitoring. This will be accompanied by its integration with microelectronics, which is also an actively studied area.
Dr Ruitao Su is a postdoctoral associate in the Department of Mechanical Engineering at the University of Minnesota. His research focuses on microfluidics and optoelectronics that are fabricated with 3D printing. His work on polymer photodetectors were selected by National Geographic as “one of the 12 innovations that will revolutionise the future of medicine” in 2018. He was the recipient of the Material Research Society (MRS) Graduate Student Silver Award in 2018 and one MRS Best Presentation Award in 2020.
Related topics
Drug Development, Drug Discovery, Lab Automation, Microfluidic Technology, Screening
Related organisations
University of Minnesota
Related people
Professor Michael McAlpine