Concerns for future gene therapy testing in laboratory mice
Posted: 7 July 2022 | Ria Kakkad (Drug Target Review) | No comments yet
Researchers have found that new sickle cell disease gene therapies depend on choosing the right laboratory mice.
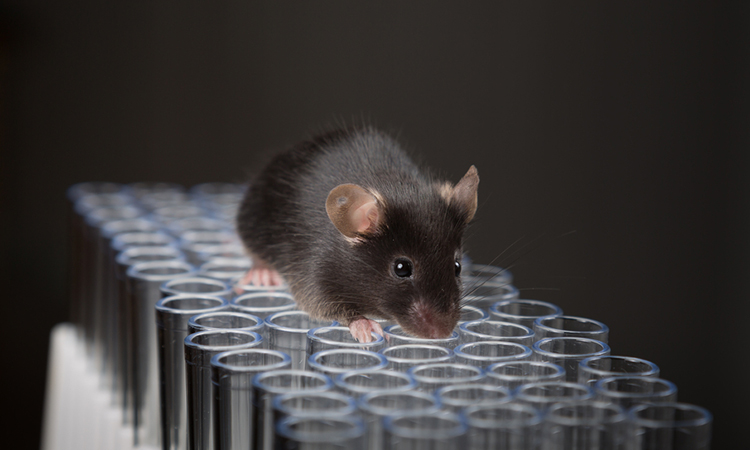
Scientists from St. Jude Children’s Research Hospital, US, have been investigating a promising new sickle cell treatment by editing genes to switch on the production of healthy, foetal haemoglobin in adult red blood cells. However, when testing the treatment in mice, the researchers found that even though the lab mice had the symptoms of sickle cell disease, the foetal haemoglobin gene and surrounding DNA were not properly configured, making the revolutionary stem-cell treatment ineffective or even harmful in the animals and raising concerns for future research testing new gene-based therapies in these laboratory mice. Their findings were recently published in Disease Models & Mechanisms.
The researchers tried their new gene therapy in two types of mice that carry the symptoms of sickle cell disease: so-called ‘Berkeley’ and ‘Townes’ mice. First, they removed stem cells from the mice and used gene editing to modify part of the stem cells’ DNA to switch on the healthy foetal haemoglobin gene. The scientists then put these reprogrammed stem cells back into the mice and monitored the animals for 18 weeks to find out how the treatment affected them.
70 percent of the Berkeley mice died from the therapy, and it only activated production of the healing foetal haemoglobin gene in 3.1 percent of mouse’s stem cells. In contrast, the experimental treatment activated the foetal haemoglobin gene in 57 percent of red blood cells in the Townes mice and did not affect the animals’ survival. However, the levels of foetal haemoglobin produced in the red blood cells of Townes mice were seven to 10 times lower than seen when this approach is used in human cells grown in the laboratory and not high enough to reduce clinical signs of sickle cell disease.
The researchers then wanted to find out why this new treatment was not successful in the Berkeley mice, which have been used for decades to test treatments for sickle cell disease, but the team did not know enough about the genetic configurations of these mice. Therefore, they sequenced the haemoglobin genes and surrounding DNA of the Berkeley mice and discovered that instead of having a single copy of the mutated human gene, the mice had 22 randomly arranged, broken-up copies of the mutated human sickle cell disease gene and 27 copies of the human foetal haemoglobin that the team had hoped to activate to cure the mice of the disease. This complex genetic make-up caused the fatal effects when the scientists tested the gene therapy in the Berkeley mice, as editing multiple copies of a gene can damage the DNA. This means that researchers cannot use these mice to test and optimise this gene-editing treatment.
In contrast, the Townes mice only had single copies of the mutated human haemoglobin gene and the gene that makes human foetal haemoglobin. However, these mice likely lacked crucial pieces of DNA that normally regulate the production of the foetal haemoglobin gene in humans. Therefore, they couldn’t produce enough of this healthy protein to alleviate the mouse symptoms.
Dr Mitchell Weiss concluded, “Our findings will help scientists using the Berkeley and Townes mice decide which to use to address their specific research question relating to sickle cell disease or haemoglobin. Additionally, this work provides a reminder for scientists to carefully consider the genetics of the mice that they are using to study human diseases and find the right mouse for the job.”
Related topics
Disease Research, Gene Testing, Gene Therapy, Genome Editing
Related conditions
sickle cell disease
Related organisations
St Jude Children’s Research Hospital
Related people
Dr Mitchell Weiss